Asteroid That Ended the Dinosaurs: Scientists Discover Its Origin
Summary
- Chicxulub Impact: An asteroid impact 66 million years ago led to the mass extinction of dinosaurs.
- Carbonaceous Asteroid: New evidence suggests the asteroid was a rare carbonaceous (C-type) asteroid.
- Outer Solar System Origin: The asteroid likely came from beyond Jupiter, in the outer solar system.
- Ruthenium Isotopes: Researchers found rare ruthenium isotopes at the K-Pg boundary, indicating a carbonaceous asteroid.
- Global Impact Layer: The debris from the impact formed a layer found in geological records worldwide.
- Mass Extinction: The impact caused drastic climate changes, leading to the extinction of 75% of Earth’s species.
- Scientific Confirmation: The presence of ruthenium serves as strong evidence of the asteroid’s carbonaceous nature.
- Further Research: The findings open new questions about asteroid origins and Earth’s history.
The Asteroid That Ended the Dinosaurs: Scientists Discover Its Origin
Once upon a time, dinosaurs roamed the Earth as the dominant species. These magnificent creatures thrived for millions of years until a catastrophic event 66 million years ago changed everything. A colossal asteroid slammed into the Earth, creating what is now known as the Chicxulub crater in present-day Mexico. This impact triggered a mass extinction event, wiping out nearly 75% of Earth’s species, including the non-avian dinosaurs. Despite extensive research, the exact nature and origin of the asteroid that caused this extinction remained a mystery—until now.
Recent research published in the journal Science has shed new light on the origin of the Chicxulub impactor. Scientists have identified that the asteroid was likely a rare carbonaceous asteroid, or C-type asteroid, originating from the outer regions of our solar system. This discovery not only helps us understand the event that ended the reign of the dinosaurs but also provides insights into the dynamics of our solar system and the potential threats that still loom.
The Chicxulub impact was a crucial event in Earth’s history. An asteroid, around 10 kilometers wide, struck with the power of billions of atomic bombs. The impact destroyed everything nearby and sent shockwaves around the world. The explosion threw huge amounts of debris into the air. This debris blocked sunlight, causing darkness on Earth. The “impact winter” that followed caused temperatures to drop sharply. This sudden cold disrupted the climate and led to the destruction of ecosystems.
This catastrophic event created the Cretaceous-Paleogene (K-Pg) boundary, a geological marker found in rock layers around the world. This boundary marks the end of the Cretaceous period and the beginning of the Paleogene period, a time when dinosaurs and countless other species perished, making way for the rise of mammals and, eventually, humans.
For decades, scientists have debated the type of asteroid that struck Earth and caused the mass extinction. Was it a common siliceous (S-type) asteroid from the inner asteroid belt, or a rare carbonaceous (C-type) asteroid from the outer solar system? The answer to this question has significant implications for understanding the risks posed by different types of asteroids.
Dr. Mario Fischer-Gödde of the University of Cologne, Germany, and his team took on this challenge. By analyzing the chemical composition of the K-Pg boundary, they found crucial evidence that points to a carbonaceous asteroid. The key to their discovery lies in the detection of ruthenium isotopes, a rare element on Earth but abundant in certain types of asteroids.
Ruthenium is one of the platinum group metals, which are extremely rare on Earth’s crust but can be found in certain types of meteorites. By studying the isotopic composition of ruthenium in the K-Pg boundary layer, the researchers discovered that the isotopes matched those found in carbonaceous chondrites— a type of carbonaceous asteroid. This discovery was a game-changer in the scientific community.
This evidence not only confirms the nature of the asteroid but also suggests that it came from the outer regions of the solar system, beyond Jupiter, where carbonaceous asteroids are more common. These asteroids are rich in carbon and water, distinguishing them from the siliceous asteroids that dominate the inner asteroid belt.
Table 1: Comparison Between S-type and C-type Asteroids
Feature | S-type Asteroids | C-type Asteroids |
---|---|---|
Composition | Silicate, Nickel-Iron | Carbon, Water, Organic Compounds |
Location in Solar System | Inner Solar System (within Jupiter’s orbit) | Outer Solar System (beyond Jupiter’s orbit) |
Frequency of Impact with Earth | Higher | Lower |
Rarity on Earth | Common | Rare |
The Chicxulub crater, with a diameter of about 150 kilometers, is one of the largest impact craters on Earth. It is located on the Yucatán Peninsula in Mexico and is partially submerged under the Gulf of Mexico. The discovery of this crater in the late 20th century provided the first solid evidence of an impact event coinciding with the extinction of the dinosaurs.
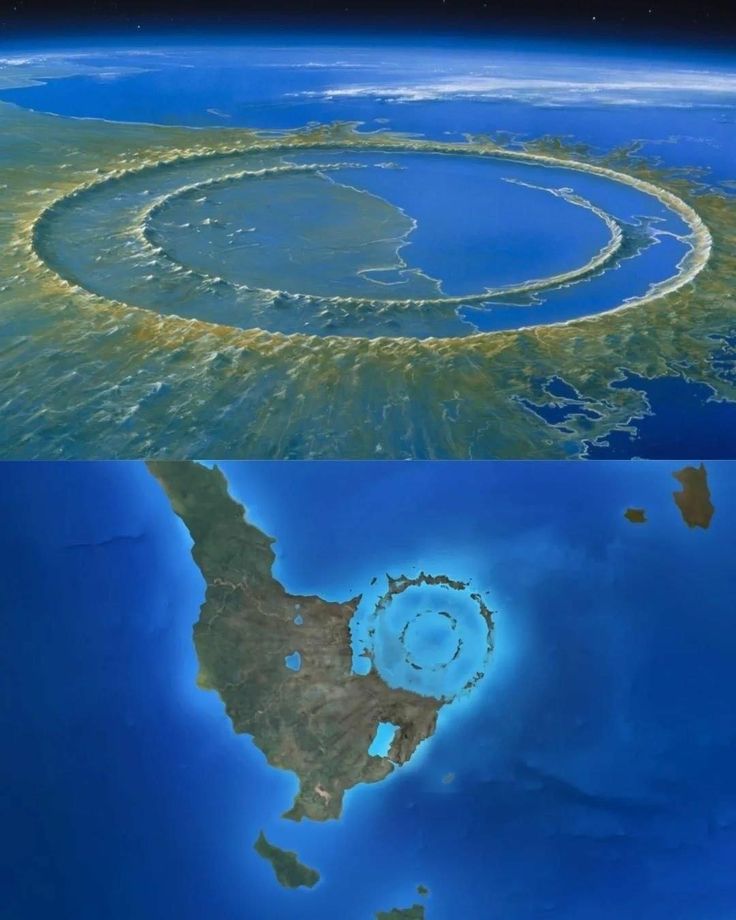
But the impact was more than just a crater. The force of the collision vaporized the asteroid and sent superheated material raining down across the planet. Massive wildfires ignited, and the atmosphere became filled with sulfuric aerosols and soot, which blocked sunlight for months, if not years. The sudden cooling, known as an “impact winter,” devastated plant life, which in turn caused a collapse in the food chain. This chain reaction led to the extinction of about 75% of all species, including the mighty dinosaurs.
The evidence of the Chicxulub impact is not limited to the crater itself. The K-Pg boundary is a thin layer of sediment found in geological formations around the world. This layer contains high concentrations of iridium, an element that is rare on Earth but common in asteroids. The presence of iridium at the K-Pg boundary was one of the first clues that an asteroid impact might have caused the mass extinction.
In addition to iridium, the layer contains shocked quartz, tektites, and microkrystites, all of which are indicators of a high-energy impact event. The layer has been found in locations as diverse as North America, Europe, Asia, and Africa, providing global evidence of the catastrophe.
Table 2: Key Findings at the K-Pg Boundary
Evidence | Description | Significance |
---|---|---|
Iridium Anomaly | High levels of iridium in the K-Pg boundary layer | Indicates extraterrestrial origin |
Shocked Quartz | Quartz grains with unique deformation patterns | Evidence of high-energy impact |
Tektites and Microkrystites | Glassy spherules formed by vaporized rock | Formed by the intense heat of impact |
Ruthenium Isotopes | Isotopic signature matching carbonaceous asteroids | Confirms asteroid type and origin |
The discovery of ruthenium isotopes at the K-Pg boundary is a significant advancement in understanding the nature of the Chicxulub impactor. Carbonaceous asteroids, or C-type asteroids, are among the most ancient objects in the solar system. They are believed to have formed in the early solar system, far from the Sun, and have remained largely unchanged since then.
These asteroids are rich in organic compounds and water, which has led some scientists to speculate that they may have played a role in delivering the building blocks of life to Earth. However, in the case of the Chicxulub impactor, the consequences were far more destructive.
The carbonaceous nature of the asteroid also explains the presence of certain rare elements, like ruthenium, in the K-Pg boundary. These elements are not commonly found on Earth, but their abundance in carbonaceous chondrites matches what has been discovered in the geological record.
The immediate aftermath of the Chicxulub impact was catastrophic. The impact winter caused by the debris and aerosols in the atmosphere led to a dramatic drop in global temperatures. Photosynthesis was severely disrupted, leading to the collapse of ecosystems. Plants died off, and with them, the herbivores that depended on them. Carnivores, in turn, lost their prey. The food chain was shattered, and many species, unable to adapt, went extinct.
This mass extinction, known as the Cretaceous-Paleogene extinction event, marked the end of the Mesozoic Era, often called the Age of Reptiles. With the dinosaurs gone, mammals, which had previously lived in the shadow of the giant reptiles, began to thrive. This event set the stage for the rise of mammals, and ultimately, the evolution of humans.
Hashtags
#ChicxulubImpact, #DinosaurExtinction, #CarbonaceousAsteroid, #CTypeAsteroid, #OuterSolarSystem, #RutheniumIsotopes, #KPgBoundary, #MassExtinction, #EarthHistory, #SpaceScience