Scientists Reveal Why Martian Soil is Extra Crusty
Recent findings from NASA’s InSight mission reveal that Martian soil is hardened by salty films, formed due to temperature changes on Mars. These crusty layers are vital to understanding the soil’s composition, which affects heat flow and could influence potential microbial life.
Summary
- InSight mission on Mars provided new insights into Martian soil through the Heat Flow and Physical Properties Package (HP3), or “Mars Mole.”
- The HP3 instrument, though limited in depth, analyzed thermal properties in the Martian soil, highlighting why it is so hard to penetrate.
- Researchers discovered that temperature cycles on Mars create salt films, leading to a crusty layer in the soil.
- This crusty layer (duricrust) is located just beneath the surface, affecting heat flow and soil properties.
- Thermal measurements showed that the soil density near the surface is comparable to basaltic sand.
- Findings may impact future Mars missions, as they indicate a level of insulation in the soil that could influence temperature-sensitive processes.
- Temperature variations near the surface could enable the formation of salty brines, which has implications for the survival of microbial life.
- The duricrust could pose challenges for exploration tools meant to dig beneath Mars’s surface.
- Insights into Martian soil contribute to theories on Mars’s geological history and heat retention.
- Understanding Martian soil could support future missions to Mars, including potential human exploration.
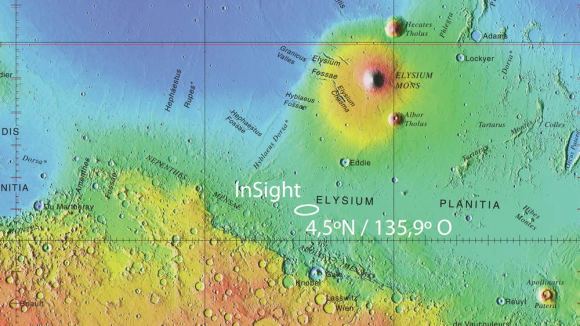
Introduction: Understanding the Martian Soil
Mars, the Red Planet, has long fascinated scientists and explorers. With its barren surface and extreme conditions, Mars is a challenging environment for exploration. NASA’s InSight mission, launched in 2018, marked a significant achievement by placing a research station on Mars dedicated to studying its subsurface. Equipped with advanced instruments, InSight aimed to collect data on Mars’s interior and provide insight into the planet’s geologic activity.
One of the primary tools used by InSight is the Heat Flow and Physical Properties Package (HP3), also known as the Mars Mole, developed by the German Aerospace Center (DLR). HP3’s objective was to dig deep into the Martian surface and measure heat flow from inside the planet, which would aid in understanding Mars’s thermal properties. Despite the unexpected difficulties faced by HP3 in penetrating the surface, scientists gathered valuable data, unveiling new insights into Martian soil’s unique properties.
Key Discoveries from the HP3 Mars Mole
The HP3 probe was designed to dig as deep as five meters, but it struggled to reach more than a few centimeters below the surface. Instead of reaching its intended depth, it managed to burrow only 40 cm (about 16 inches) into the soil. This limitation, however, yielded a surprising discovery about the Martian surface: a crusty layer formed by salty brines hardened the soil.
Thermal Properties of Martian Soil
The data collected by HP3 allowed scientists to analyze thermal conductivity and soil density on Mars. By comparing subsurface temperatures recorded by InSight with surface temperatures, scientists measured the thermal diffusivity and thermal conductivity of Martian soil. This data has been crucial for understanding Mars’s thermal environment.
“The thermal conductivity data we obtained provided a valuable look into the physical properties of Martian soil, even though we were unable to dig as deep as originally intended,” explained Tilman Spohn, Principal Investigator for the HP3 experiment at the DLR Institute of Planetary Research.
Why is Martian Soil So Crusty?
1. Formation of Salt Films in Martian Soil
The research conducted by the DLR team shows that temperature fluctuations in the top 40 cm of Mars’s surface lead to the formation of salt films. These salty films, formed when there’s enough moisture, harden the soil and create a crust-like layer. This encrusted soil, also called duricrust, likely consists of salty brines solidifying beneath the surface during cold Martian nights.
2. Seasonal and Daily Temperature Cycles
On Mars, surface temperatures fluctuate significantly due to its thin atmosphere and distant position from the Sun. During the day, temperatures can rise dramatically, only to plummet at night. According to data, Martian soil temperatures just below the surface shift between -56°C and -60°C daily. Although temperature cycles impact surface and near-surface soil, they stabilize at greater depths, leading to variations that encourage brine formation.
Measurement | Temperature (°C) | Temperature (°F) |
---|---|---|
Daytime Surface Temperature | -56 | -68.8 |
Nighttime Surface Temperature | -60 | -76 |
Average Near-Surface Temperature | -58 | -72.4 |
These temperature shifts cause salts in the soil to absorb moisture from the atmosphere, forming brine during specific seasons. The brine subsequently hardens, creating a crusty surface layer resistant to digging and drilling.
Martian Soil’s Composition and Density
The soil density on Mars’s surface layer has surprised scientists. By comparing HP3’s measurements with known earth materials, researchers deduced that the top 30 cm (~12 inches) of soil resemble basaltic sand, which commonly forms through volcanic activity. Beneath this layer lies a denser, more consolidated soil, likely made of coarse basalt fragments.
Martian Soil Depth | Material Density | Comparison |
---|---|---|
0-30 cm (~12 in) | Basaltic Sand | Similar to Earth’s sand |
30-50 cm (~20 in) | Consolidated Coarse Fragments | Harder, resistant layer |
This stratification affects how heat is transferred and stored, which could play a key role in the stability and behavior of Martian soil, especially when considering its interaction with temperature cycles and potential drilling operations for future Mars missions.
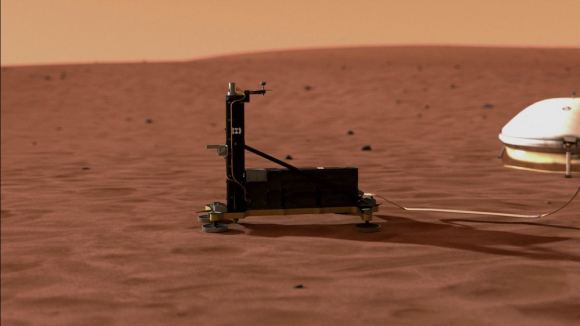
Implications for Future Mars Missions
1. Geological Activity and Thermal Insulation
The Martian soil’s crusty layer acts as an insulator, moderating temperature fluctuations below the surface. This insulation could suggest that Mars retains some geological activity, although at a much slower rate than Earth. With these findings, scientists believe that the Martian core may still possess a degree of thermal activity.
2. Potential for Microbial Life
The crusty soil layer may also impact any search for microbial life. The formation of salty brines near the surface provides an environment where life, if it exists, could potentially survive. Even with extreme surface conditions, the protected soil layer may contain the right conditions for microbial life, especially if future missions discover water or hydrated minerals.
“Temperature has a strong influence on chemical reactions occurring in the soil, on the exchange with gas molecules in the atmosphere, and therefore also on potential biological processes regarding possible microbial life on Mars,” said Spohn, highlighting the relevance of these findings.
3. Soil Hardness and Exploration Challenges
The crusty layer poses a technical challenge for drilling and sampling tools on Mars. As HP3 demonstrated, penetrating the duricrust layer requires tools equipped to handle hardened soil. Future missions to Mars will need to develop more advanced tools that can break through this crust and access deeper layers. Insights from HP3’s challenges could lead to more effective drilling technology for human missions.
4. Scientific Implications for Mars’s Geological History
The duricrust layer offers a window into Mars’s past. Scientists speculate that Mars’s geological activity may have significantly diminished during the Hesperian period, about 3 billion years ago. This period is characterized by reduced volcanic activity and cooling of the Martian core. Evidence from the HP3 data supports theories that Mars’s outer core solidified due to its smaller size and mass compared to Earth, potentially impacting the planet’s geological evolution and surface conditions.
Facts About Mars’s Crusty Soil
- The duricrust layer on Mars might extend to about 20 cm (~8 inches) beneath the surface, hardened by salty brines that form seasonally.
- Unlike Earth, Mars lacks an ozone layer, so UV radiation can penetrate the surface. This might affect the soil’s chemical composition.
- Basaltic sand on Mars, found near the surface, is similar to volcanic sand on Earth, possibly formed from ancient volcanic activity.
- Due to Mars’s thin atmosphere, temperature variations are extreme, but the soil’s crusty layer helps stabilize temperatures beneath the surface.
- The crusty layer of soil could be an indicator of past hydrological activity on Mars, pointing to water’s role in shaping the planet’s surface.
NASA’s InSight mission has provided valuable data that reshapes our understanding of Martian soil. The discovery of the crusty duricrust layer, formed by salty films, reveals how temperature cycles shape Mars’s surface. While the HP3 instrument faced challenges, its findings are crucial for future Mars exploration, offering insights into the challenges posed by the Martian soil.
Understanding Martian soil’s density, thermal properties, and insulating capabilities will be vital for future missions, especially those involving drilling or human exploration. As scientists continue to analyze data from the InSight mission, they may uncover even more about Mars’s geological history, surface conditions, and the planet’s potential to support life.
References
- NASA’s InSight Mission Overview
- German Aerospace Center (DLR) News
- HP3 Instrument Details
- DLR Space Operations and Astronaut Training