Extremely Large Telescope: Detecting Hints of Life at Proxima Centauri Within 10 Hours
The upcoming Extremely Large Telescope (ELT) will revolutionize our view of the universe by capturing incredibly detailed images and spectra from exoplanet atmospheres. With its enormous 39‑meter mirror and advanced technology, the ELT is expected to detect key chemical signatures—such as water, carbon dioxide, and oxygen—that may indicate the presence of life around nearby stars like Proxima Centauri in as little as ten hours of observation.
Summary
- Breakthrough capability: The ELT’s 39‑meter mirror collects light at an unprecedented scale.
- Sharper images: Produces images 16 times sharper than those from the Hubble Space Telescope.
- Exoplanet insights: Studies both transiting and non‑transiting exoplanets via spectral analysis.
- Life detection: Simulations suggest the possibility of detecting life on Earth‑like worlds near Proxima Centauri.
- Advanced technology: Uses adaptive optics and state‑of‑the‑art sensors to overcome Earth’s atmospheric distortions.
- Wide impact: Its discoveries could answer long‑standing questions about extraterrestrial life.
- Collaborative research: Involves international teams and multidisciplinary research efforts.
- Technological leap: Represents a significant advancement over previous telescopes like JWST.
- Astrobiological promise: Provides new methods to study planetary habitability and atmospheric composition.
- Enhanced sensitivity: Capable of analyzing faint spectral lines that indicate the presence of key molecules.
- Simulated scenarios: Recent studies simulate various Earth‑like atmospheres to test the ELT’s effectiveness.
- Scientific milestone: Marks the dawn of a new era in observational astronomy.
- Innovative design: Combines revolutionary optics with powerful computational methods.
- Global interest: Promises to influence future space exploration and scientific research worldwide.
- Historical significance: A step that may finally help answer the question, “Are we alone?”
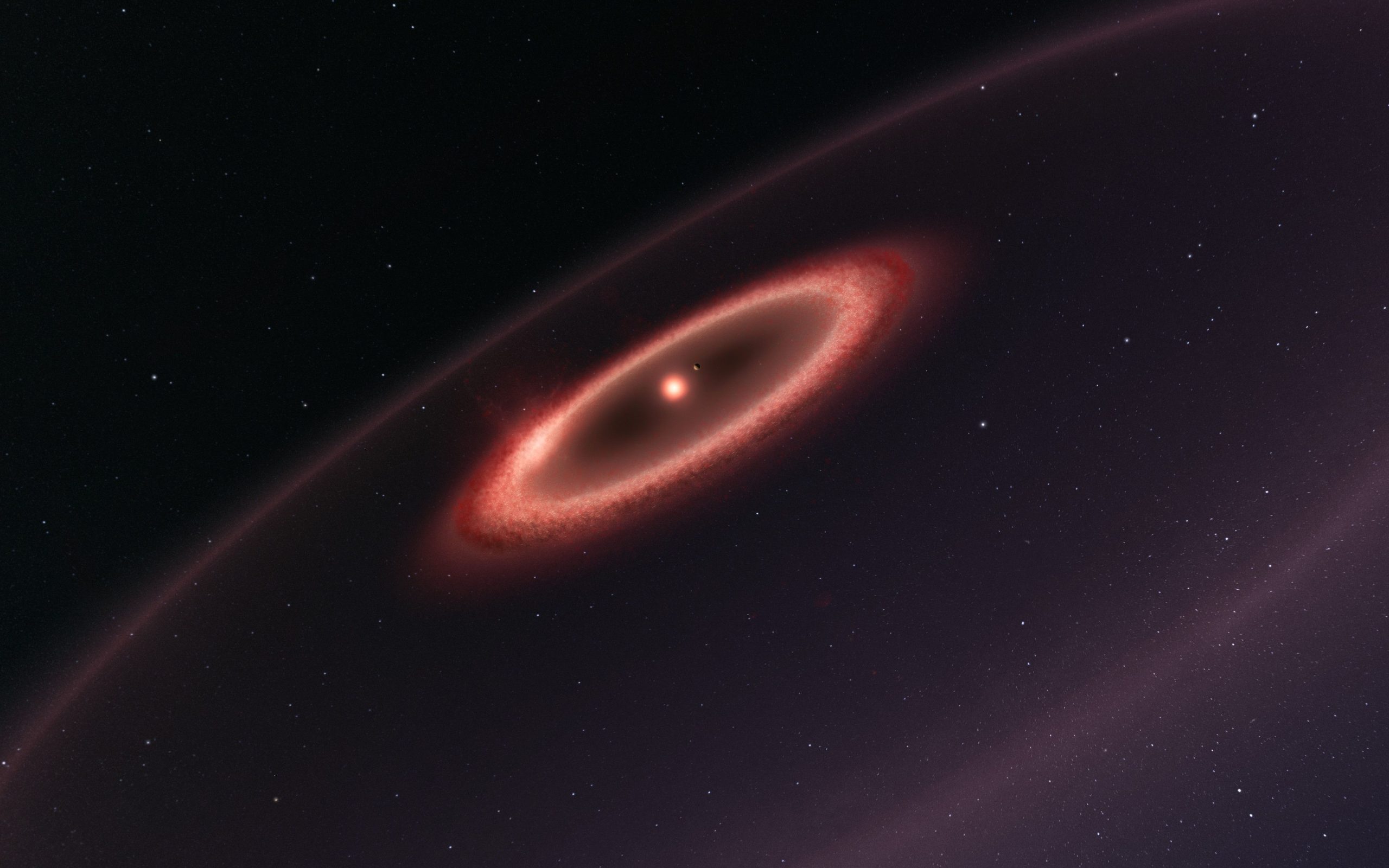
Introduction
The Extremely Large Telescope (ELT) is a groundbreaking project under construction in northern Chile. Designed to push the boundaries of observational astronomy, the ELT’s 39‑meter primary mirror will collect far more light than any previous ground‑based telescope. This immense capability will enable scientists to obtain images and spectra with an unprecedented level of detail. With the potential to detect atmospheric molecules in exoplanets, the ELT promises to be an indispensable tool in our search for extraterrestrial life. Its design and technology combine modern engineering with innovative astronomical techniques, ensuring that every photon captured leads us closer to understanding the cosmos.
Understanding the ELT
The ELT is engineered to overcome the limitations of earlier telescopes by gathering and analyzing starlight that interacts with distant exoplanet atmospheres. When a planet passes in front of its star, a small portion of the star’s light filters through the planet’s atmosphere. This filtered light carries the signatures of various molecules. By examining these absorption features, scientists can deduce the atmospheric composition and even infer the presence of life. Unlike previous missions, the ELT’s superior light‑gathering power means that even the faintest spectral lines can be observed. Its ability to capture such delicate details is a tremendous leap forward from the capabilities of telescopes like the Hubble Space Telescope or the James Webb Space Telescope.
Exoplanet Exploration Techniques
Traditional methods of exoplanet study rely heavily on transit observations, where a planet crosses in front of its host star. However, many exoplanets do not transit their stars from our line of sight. The ELT will extend our reach by also examining reflected starlight from these non‑transiting planets. This approach broadens the range of targets available for study, making it possible to analyze a greater variety of planetary atmospheres. With this method, even planets that have been elusive to other instruments can now be scrutinized for signs of water, oxygen, and other life‑supporting molecules. The integration of multiple observation techniques ensures that the ELT will offer a comprehensive view of the diverse worlds beyond our solar system.
Simulation Studies and Test Cases
Recent simulation studies have been conducted to assess the ELT’s capabilities across various planetary scenarios. Researchers considered several test cases, ranging from a water‑rich, non‑industrial Earth to a pre‑biotic Earth that shows no evidence of life. The results of these simulations are summarized in the tables below.
Scenario | Description | Observation Time |
---|---|---|
Non‑industrial Earth | An Earth‑like planet with abundant water and thriving photosynthetic life. | Approximately 10 hours |
Early Archean Earth | A young Earth where primitive life is just beginning to develop. | Approximately 10 hours |
Evaporated Ocean Earth | A planet that has lost its water, resembling conditions on Mars or Venus. | Approximately 10 hours |
Pre‑biotic Earth | A potentially habitable world that currently shows no biological activity. | Approximately 10 hours |
Neptune‑sized World | A larger planet with a thick, extensive atmosphere. | Approximately 1 hour |
Telescope | Light Gathering Power | Image Sharpness | Observation Efficiency |
---|---|---|---|
Hubble Space Telescope | Moderate | Good | Low |
James Webb Space Telescope | High | Excellent | Moderate |
Extremely Large Telescope | Extremely High | Superior | Very High |
These tables demonstrate that the ELT not only surpasses its predecessors in terms of light‑collecting power but also in its ability to produce clear and detailed images. The simulations indicate that, for the closest star systems, the ELT could detect biosignatures in an Earth‑like atmosphere in as little as ten hours of observation.
Inspirational Reflection
In the middle of our journey through the stars, it is important to remember that our quest for knowledge is also a quest for self‑understanding. “The cosmos is within us. We are made of star‑stuff.” This profound thought encourages us to explore the universe with curiosity and humility, knowing that every discovery brings us closer to understanding the essence of life itself.
Technological Innovations
The ELT incorporates a range of cutting‑edge technologies. Its adaptive optics system actively compensates for the Earth’s turbulent atmosphere, ensuring that the light collected is as clear as possible. This real‑time correction makes it possible to resolve incredibly fine details in distant objects. Additionally, the telescope employs advanced sensors and imaging systems that work together to process the massive amounts of data gathered during observations. These technological innovations are what set the ELT apart from previous instruments, making it a true marvel of modern science.
Implications for Astrobiology
One of the most exciting prospects of the ELT is its potential contribution to astrobiology. By detecting atmospheric molecules that are typically associated with life, the telescope might be able to provide the first evidence of life beyond Earth. For example, the presence of water vapor, oxygen, and carbon dioxide in the atmosphere of an exoplanet could be a strong indicator of biological processes. A recent study by Currie and Meadows, available on arXiv, supports the idea that the ELT could distinguish between a lifeless planet and one that harbors life. This capability is particularly promising for red dwarf stars such as Proxima Centauri, which is one of our closest stellar neighbors. More details about Proxima Centauri can be found on Wikipedia.
Future Prospects
The discoveries made by the ELT are expected to have a profound impact on our understanding of the universe. Its advanced design will not only help to identify the chemical makeup of distant atmospheres but also aid in the study of the formation and evolution of galaxies. As scientists continue to refine their techniques, the ELT’s observations may lead to the development of even more powerful telescopes in the future. International collaborations and interdisciplinary research will drive further advances in astronomy, paving the way for breakthroughs that could transform our view of the cosmos.
The Extremely Large Telescope stands as a beacon of human ingenuity and scientific progress. Its extraordinary capabilities promise to open a new chapter in our exploration of the universe. By delivering clear images and detailed spectral data, the ELT will help answer fundamental questions about the existence of life on other planets. As we look forward to its first light in 2028, the excitement builds around the possibility of discovering life around stars like Proxima Centauri in record time. This momentous achievement will not only expand our knowledge of the cosmos but also inspire future generations to continue exploring the mysteries of our universe.
Reference: Currie, Miles H., and Victoria S. Meadows. “There’s more to life in reflected light: Simulating the detectability of a range of molecules for high-contrast, high-resolution observations of non-transiting terrestrial exoplanets