Einstein’s Theory Just Survived Its Most Difficult Challenge in History
Albert Einstein’s theory of general relativity, formulated over a century ago, remains an unshaken pillar of physics even after undergoing one of its most demanding tests. A team of scientists used the Dark Energy Spectroscopic Instrument (DESI) to study nearly six million galaxies over 11 billion years. This analysis confirmed that the theory holds true across vast cosmic scales, shaping our understanding of gravity, dark matter, and dark energy.
Summary
- General relativity provides the framework for understanding gravity’s behavior in space and time.
- The Dark Energy Spectroscopic Instrument (DESI) used advanced mapping techniques to observe galaxies and quasars.
- Findings show that galactic formations and movements follow predictions of general relativity even at cosmic scales.
- The research places limits on the mass of neutrinos and probes the nature of dark matter and energy.
- This study demonstrates the precision of Einstein’s equations over 11 billion years of cosmic evolution.
- DESI will continue to gather data, mapping 40 million celestial objects by the end of its mission.
- These insights may finally solve some of the greatest mysteries in physics.
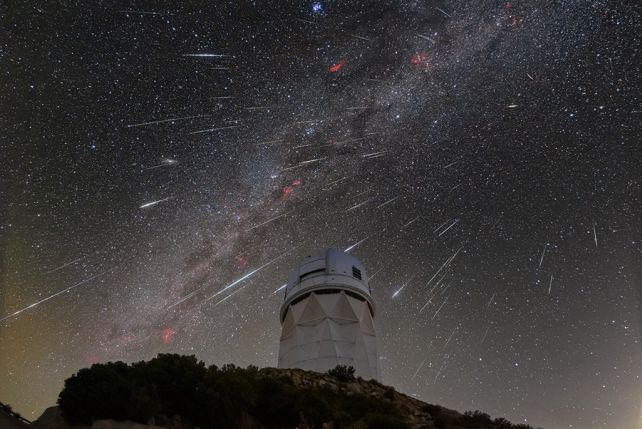
Introduction
Albert Einstein’s general relativity is one of the most profound scientific achievements of the 20th century. Its implications extend across the universe, from predicting planetary orbits to understanding black holes. But can this theory withstand the test of time? A monumental new study led by the Dark Energy Spectroscopic Instrument (DESI) indicates that it can.
By examining nearly 6 million galaxies distributed over 11 billion years of cosmic history, researchers have confirmed that the predictions made by Einstein’s equations align remarkably well with observable reality. The results are accessible online through DESI’s published findings on arXiv and related news releases.
Understanding General Relativity
Einstein’s theory describes how gravity arises from the curvature of spacetime caused by mass. Unlike earlier Newtonian concepts, general relativity explains phenomena like:
- The bending of light around massive objects (gravitational lensing).
- The precession of Mercury’s orbit.
- The warping of spacetime near black holes.
Einstein’s theory bridges the gap between quantum mechanics and classical physics. Validating or disproving it at cosmic scales could open new doors to understanding dark energy and dark matter, which collectively compose 95% of the universe.
The DESI Mission
DESI, based in Arizona at the Mayall Telescope, represents an international collaboration aimed at creating the most detailed 3D map of the universe. Its sophisticated instruments allow astronomers to study:
- Galactic distribution: How galaxies cluster along the cosmic web.
- Quasar evolution: The behavior of supermassive black holes over time.
- Dark matter influences: Mapping gravitational effects in otherwise invisible regions.
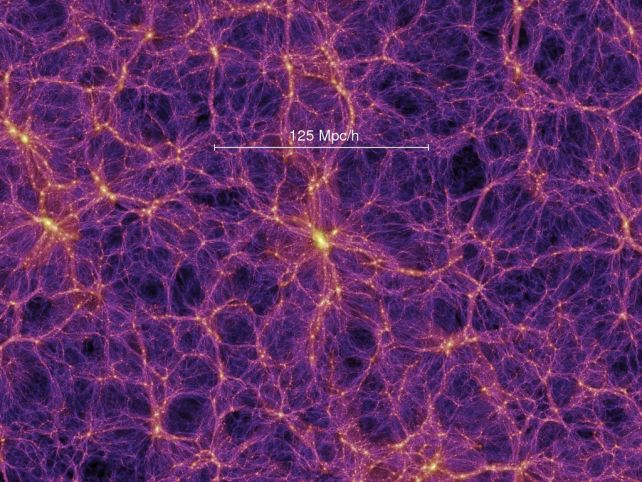
Table 1: Key DESI Observations
Observation | Findings |
---|---|
Distribution of 5.7 million galaxies | Galaxies align with predicted clustering patterns in general relativity. |
Cosmic web dynamics | Structures grow as expected under Einstein’s equations. |
Neutrino mass constraints | Study places upper limit on the mass of neutrinos. |
Expansion of the universe | Observations match models for dark energy-driven acceleration. |
Testing Gravity Across Time
The DESI team compared current galaxy distributions with predictions from 11 billion years ago, simulating alternate scenarios with stronger or weaker gravitational forces. They concluded that even slight deviations from general relativity would result in drastically different cosmic arrangements.
Simulations, like those conducted by DESI researchers Claire Lamman and Michael Rashkovetskyi, demonstrate how altering gravity changes the cosmic web structure. For more details, you can visit the DESI website.
Cosmic Mysteries: Dark Energy and Matter
Dark energy and dark matter dominate discussions of cosmic evolution.
- Dark matter: Provides extra gravitational pull, shaping galaxies and the web-like cosmic structure.
- Dark energy: Drives the universe’s accelerating expansion.
Table 2: Major Unknowns in the Universe
Phenomenon | Percentage of Universe | Current Understanding |
---|---|---|
Dark Matter | ~25% | Generates gravitational pull but remains invisible. |
Dark Energy | ~70% | Drives expansion; origin unknown. |
Normal Matter | ~5% | Includes stars, planets, and visible material. |
Future Implications
The DESI collaboration is far from finished. Researchers plan to collect data on 40 million celestial objects, offering a treasure trove of information to refine our understanding of the universe.
Advancements in general relativity testing have practical implications:
- Enhancing satellite navigation systems.
- Improving models for gravitational wave detection.
- Expanding our ability to predict cosmic phenomena.
Facts About General Relativity
- Einstein’s theory predicted black holes decades before they were observed.
- GPS systems would fail without accounting for general relativity’s effects on time.
- The concept of spacetime warping inspired countless sci-fi movies, including Interstellar.
- Einstein initially doubted his own predictions about gravitational waves!
Einstein’s general relativity continues to withstand the most challenging tests. The DESI collaboration’s groundbreaking survey not only validates his equations but also brings us closer to understanding the dark universe. As scientists gather more data, they hope to illuminate the mysterious forces shaping cosmic evolution.
The quest to solve the secrets of gravity, dark energy, and dark matter is far from over. To learn more about DESI’s ongoing mission, check their official updates.
References
- DESI Collaboration Papers
- New DESI Results Weigh in on Gravity
- Where Does Gravity Come From?
- Lawrence Berkeley National Laboratory