Curiosity Rover Discovers Fossilized Wave Ripples on Mars
NASA’s Curiosity Rover has uncovered fossilized wave ripples on Mars, providing the strongest evidence yet of open, ice-free liquid water in the planet’s ancient history. These findings suggest that Mars’ climate was once warm and dense enough to support shallow lakes 3.7 billion years ago, fundamentally reshaping our understanding of its past environment.
Summary
- The Curiosity Rover, part of NASA’s Mars Science Laboratory mission, has been exploring the planet since 2012.
- Curiosity discovered ancient wave ripples in the Gale Crater, confirming the presence of ice-free, liquid water.
- These ripples, preserved in rock, resemble patterns seen in Earth’s shallow lakebeds.
- Analysis reveals that Mars’ climate 3.7 billion years ago was warmer and denser, enabling liquid water to exist in open air.
- Two separate sites were studied: Prow outcrop and Amapari Marker Band, both showing ripples at different periods.
- The ripples were caused by wind-driven water, suggesting shallow bodies of water, less than 2 meters deep.
- Earlier discoveries by the Opportunity Rover suggested liquid water, but this finding is the clearest evidence yet.
- This discovery offers critical insights into Mars’ paleoclimate and raises the possibility of microbial life.
- More investigations are needed to determine how widespread these ripples are across the Martian surface.
- Research was led by Caltech scientists John Grotzinger and Michael Lamb.
- A detailed paper was published in Science Advances.
- The findings are pivotal for understanding the history of water on Mars and its potential for habitability.
- Mars, known as the “Red Planet,” has long intrigued scientists due to its similarities to Earth.
- The discovery adds to the growing body of evidence of Mars’ once hospitable environment.
- Curiosity’s continued mission aims to uncover more about the planet’s climate, geology, and potential for life.
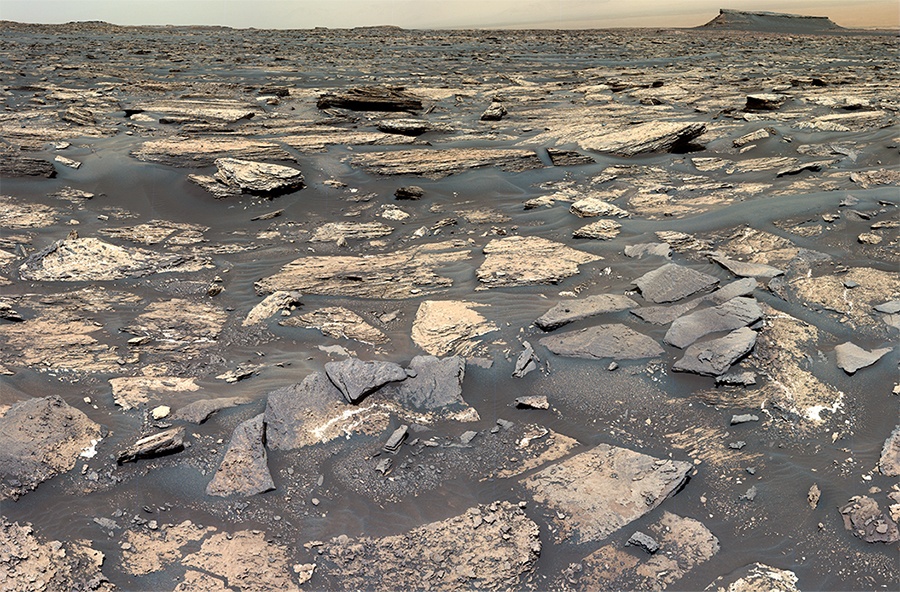
Mars: A Planet of Mysteries
Mars, the fourth planet from the Sun, has captivated humanity for centuries. Known for its reddish appearance caused by iron oxide, Mars shares some intriguing similarities with Earth, including valleys, volcanoes, and evidence of dried riverbeds. However, its thin atmosphere, unbreathable air, and extreme cold set it apart. Despite these challenges, scientists have long speculated about Mars’ potential to support life, leading to groundbreaking missions like NASA’s Curiosity Rover.
The Curiosity Rover and Its Mission
NASA’s Curiosity Rover, part of the Mars Science Laboratory mission, landed on the Red Planet in August 2012. Its primary mission is to investigate Mars’ climate and geology and assess whether the planet could have supported microbial life in the past. The rover is equipped with advanced tools, including drills, cameras, and atmospheric analyzers, allowing it to collect and analyze samples from Mars’ surface.
One of the rover’s most significant recent discoveries came from its exploration of the Gale Crater, where it identified fossilized wave ripples. These patterns, formed by wind-driven water, indicate that Mars once hosted shallow lakes exposed to open air, reshaping our understanding of the planet’s ancient environment.
Table 1: Key Instruments on the Curiosity Rover
Instrument | Function |
---|---|
ChemCam | Laser-induced breakdown spectroscopy for chemical analysis |
MAHLI | Close-up imaging of Martian rocks and soil |
SAM | Sample analysis of organic compounds and gases |
Mastcam | High-resolution imaging |
APXS | X-ray spectrometer for elemental composition |
Discovery of Ancient Wave Ripples
The fossilized wave ripples were found in two key locations within the Gale Crater: the Prow outcrop and the Amapari Marker Band. These formations, preserved in Martian rock, closely resemble ripple patterns seen on Earth’s beaches and lakebeds, where wind-driven water flows across shallow surfaces.
Scientists analyzed the ripples to determine their age and the conditions under which they formed. Their findings indicate that the ripples were created approximately 3.7 billion years ago, during a time when Mars’ climate was warm and dense enough to support open, liquid water.
“The ripples provide the strongest evidence yet that Mars once had a warm, dense atmosphere capable of sustaining shallow, ice-free lakes,” said Dr. John Grotzinger, a geologist at Caltech.
The ripple heights, measuring about 6 millimeters with separations of 4 to 5 centimeters, suggest that the lakes were shallow, likely no more than 2 meters deep. These findings provide critical insights into Mars’ paleoclimate, revealing a planet that was once far more hospitable than it is today.
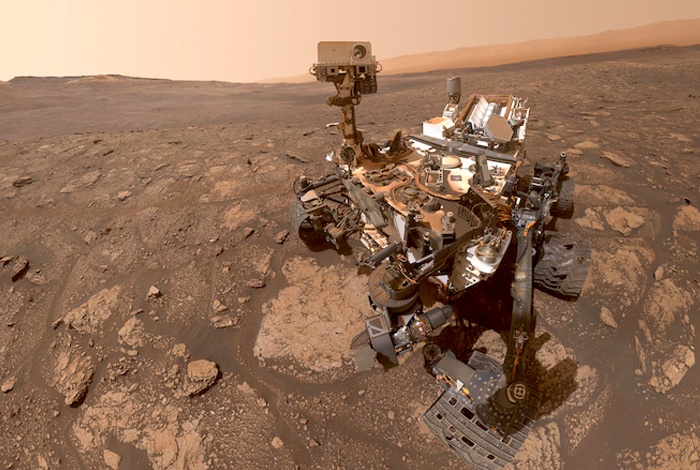
Table 2: Comparison of Martian and Earth Wave Ripples
Feature | Earth | Mars |
---|---|---|
Formation Process | Wind-driven water in shallow lakes | Wind-driven water in ancient lakes |
Ripple Height | 5-10 mm | 6 mm |
Ripple Separation | 5-8 cm | 4-5 cm |
Preservation | Temporary unless fossilized | Fossilized in rock |
Significance of the Discovery
The discovery of these ripples has far-reaching implications for our understanding of Mars’ history. Unlike previous findings, which suggested that water on Mars was frozen or subsurface, this evidence confirms the presence of liquid water exposed to the elements.
The discovery also suggests that Mars’ climate underwent significant changes over time. The presence of ripples in two distinct locations and periods indicates that the warm, dense atmosphere necessary for liquid water existed for extended periods or occurred multiple times throughout the planet’s history.
Mars’ Paleoclimate and Habitability
The findings provide invaluable data for Mars paleoclimate studies. By analyzing the size and separation of the ripples, scientists can infer details about the depth and extent of the ancient lakes. These studies are crucial for understanding how Mars transitioned from a warm, wet environment to the cold, dry planet we see today.
Moreover, the discovery raises exciting possibilities about the planet’s potential to support life. Liquid water is a key ingredient for life as we know it, and the presence of shallow, open lakes increases the likelihood that Mars may have once hosted microbial life.
Future Exploration and Research
The Curiosity Rover continues to explore the Martian surface, collecting data to build a more comprehensive picture of the planet’s history. Meanwhile, new missions, such as the Perseverance Rover and the European Space Agency’s Rosalind Franklin Rover, aim to expand on these discoveries.
Further investigations are needed to determine how widespread these fossilized ripples are and whether similar features can be found in other regions of Mars. This will help scientists understand the global extent of Mars’ ancient lakes and their role in shaping the planet’s surface.
Fun Facts About Mars
- Mars is home to the largest volcano in the solar system, Olympus Mons.
- The planet’s day is slightly longer than Earth’s, lasting 24 hours and 37 minutes.
- Mars’ thin atmosphere is composed mainly of carbon dioxide, making it unbreathable for humans.
- The planet has two moons, Phobos and Deimos, which are thought to be captured asteroids.
- Mars has been explored by more than 50 missions, including orbiters, landers, and rovers.