Hidden Structures Detected Inside Earth’s Core: What We Know So Far
Most of us do not think much about the ground beneath our feet. But it is more than just dirt or rocks. The ground has complicated layers. These layers are similar to the pages of a book. They hold Earth’s history. They also hold our history.
“Traditionally we’ve been taught the Earth has four main layers: the crust, the mantle, the outer core, and the inner core,” explained Australian National University geophysicist Joanne Stephenson in 2021. However, recent research suggests there is more to this story—Earth’s inner core may hold an even deeper secret.
Scientists have uncovered evidence that Earth’s inner core may consist of two distinct layers, challenging conventional models of Earth’s internal structure. By analyzing seismic waves and modeling their behavior, researchers have suggested that the iron crystals in the inner core have varying alignments. This discovery could rewrite our understanding of Earth’s formation and history.
Summary
- Earth is composed of four main layers: crust, mantle, outer core, and inner core.
- Research by geophysicists suggests the inner core may have two distinct layers.
- The study analyzed seismic waves traveling through Earth’s layers to uncover these findings.
- Differences in seismic wave speeds suggest variations in the core’s material structure.
- The inner core, composed mainly of iron, may have different crystal alignments in its deepest region.
- This discovery indicates the possibility of two distinct cooling events in Earth’s history.
- Previous studies hinted at this innermost core, but definitive evidence was lacking until recently.
- Global seismic data, including earthquake records, were used in the study.
- Researchers note data gaps, particularly at polar antipodes, limit the accuracy of their findings.
- Future studies aim to refine these observations and explore the implications for Earth’s history.
- This discovery challenges current textbooks and models of Earth’s interior.
- It adds a piece to the puzzle of Earth’s formation and the dynamics of its internal processes.
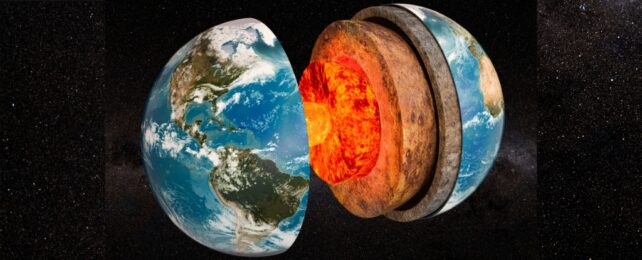
Understanding Earth’s Layers
Traditionally, Earth’s structure has been divided into four layers:
1. Crust: The outermost layer, where we live, composed of solid rock.
2. Mantle: A thick layer of semi-solid rock that moves slowly over time.
3. Outer Core: A liquid layer composed mainly of molten iron and nickel.
4. Inner Core: A solid sphere made primarily of iron, surrounded by the outer core.
However, this model may now need revision. Seismic waves, generated by earthquakes, have provided the primary tool for understanding Earth’s internal structure. These waves travel at different speeds depending on the material they pass through, revealing critical insights about the composition and properties of Earth’s layers.
A Closer Look at the Inner Core
The inner core, long thought to be a homogenous solid layer, has been a subject of fascination for geologists and geophysicists. Recent studies have shown that this layer might not be as uniform as previously believed. Instead, it may have two distinct sub-layers with varying properties.
This groundbreaking research was led by Joanne Stephenson and her team at the Australian National University, using data collected by the International Seismological Centre. The team analyzed seismic waves traveling through the inner core and found evidence suggesting a change in the structure of iron crystals.
Evidence of a Hidden Layer
By examining the anisotropy of seismic waves—how their speed changes depending on direction—the researchers discovered differences in the core’s composition.
- Some models suggest seismic waves travel faster parallel to Earth’s rotational axis.
- Others propose a distinct angle of 54 degrees where slower wave speeds occur, hinting at differences in material properties.
These findings align with earlier studies but provide more robust evidence for the presence of a hidden layer within the inner core.
“We found evidence that may indicate a change in the structure of iron, which suggests perhaps two separate cooling events in Earth’s history,” Stephenson noted in her paper. “The details of this big event are still a bit of a mystery, but we’ve added another piece of the puzzle when it comes to our knowledge of the Earth’s inner core.”
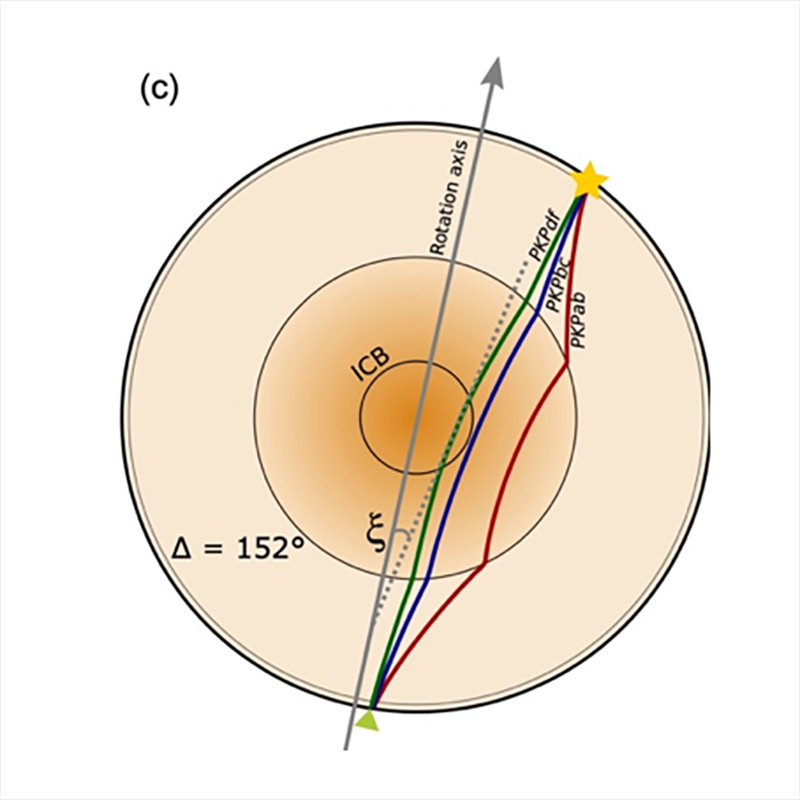
Implications for Earth’s History
This discovery has profound implications for our understanding of Earth’s history and its dynamic processes.
- Cooling Events:
The presence of a second layer within the inner core suggests two distinct cooling events in Earth’s past. These events may have shaped the planet’s magnetic field and its ability to sustain life. - Iron Crystal Alignment:
Variations in iron crystal alignments within the core could explain inconsistencies in seismic data and provide clues about the Earth’s formation. - Revising Models:
Current models of Earth’s internal structure may need to be revised to incorporate these findings, leading to a deeper understanding of planetary dynamics.
Table 1: Key Differences Between Earth’s Inner Core Layers
Property | Traditional Inner Core | New Hidden Layer (Innermost Core) |
---|---|---|
Composition | Solid iron-nickel | Differing iron crystal alignments |
Seismic Wave Anisotropy | Parallel to equator | Parallel to rotational axis |
Discovery | Long known | Recent findings by seismic studies |
Challenges in Studying the Core
Despite these groundbreaking discoveries, significant challenges remain in studying Earth’s inner core.
Data Limitations
The researchers noted gaps in seismic data, particularly at polar antipodes, which limit the certainty of their conclusions. Improved seismic networks and better data collection techniques will be essential for refining these findings.
Future Research
Future studies aim to:
- Fill in data gaps and improve the accuracy of seismic models.
- Explore the implications of the innermost core for Earth’s magnetic field.
- Investigate how these findings relate to other planetary bodies with similar structures.
Table 2: Tools for Studying Earth’s Core
Method | Description | Example Application |
---|---|---|
Seismic Waves | Analyze wave speeds and directions to infer material properties | Understanding anisotropy in the inner core |
Computer Modeling | Simulate core conditions to test hypotheses | Modeling iron crystal behavior |
Volcanic Rock Analysis | Study materials brought to the surface by volcanic activity | Identifying core-mantle boundary composition |
The Bigger Picture
Understanding Earth’s inner core is not just an academic exercise. It has practical implications for understanding the dynamics of our planet, including:
- Magnetic Field Generation: The core’s composition and movement drive Earth’s magnetic field, which protects life from harmful solar radiation.
- Geological Activity: Core processes influence volcanic activity, plate tectonics, and earthquake generation.
- Planetary Comparisons: Studying Earth’s core can help us understand the interiors of other planets, such as Mars and Venus.
Fun Facts
- The inner core’s temperature exceeds 5,000 degrees Celsius, hotter than the surface of the Sun.
- It is roughly the size of the Moon but has a density similar to pure iron.
- Seismic waves can travel through the inner core in just a few minutes, providing valuable data for scientists.
References
- Scientists Dig Deep to Reveal Earth’s Hidden Layer
- Journal of Geophysical Research: Solid Earth
- Seismic Wave Anisotropy