Earth Bombarded by Moon Debris: 1 in 4 Ejecta Makes the Trip
A recent high-precision simulation study finds that 22.6% of material blasted off the Moon by impacts eventually collides with Earth—half of those impacts happen within the first 10,000 years. This work reveals new patterns in how lunar debris travels through space, showing an equatorial concentration on Earth, a strong dependence on where on the Moon the debris was launched, and a small but measurable contribution of lunar fragments to the near-Earth object population. These insights reshape our understanding of Earth–Moon material exchange and have implications for planetary science, meteoroid hazard assessment, and the origin of certain near-Earth asteroids.
Summary
- The Moon’s surface preserves billions of years of impact history due to its lack of atmosphere and geological reshaping.
- Large impacts can eject material at speeds exceeding lunar escape velocity (2.38 km/s).
- Researchers used the REBOUND N-body simulation package with the IAS15 integrator to track 6,000 test particles for 100,000 years.
- Ejecta fragments modeled were tens of meters in size, reflecting realistic crater-forming impacts.
- 22.6% of all simulated lunar ejecta fragments collided with Earth over the 100,000-year timeframe.
- Half of those collisions occurred within the first 10,000 years after ejection.
- The collision rate follows a power-law decay C(t)∝t0.315C(t)\propto t^{0.315}.
- Debris launched from the Moon’s trailing hemisphere has the highest probability of hitting Earth.
- Fragments from the leading hemisphere are least likely to reach Earth.
- Impact speeds upon Earth arrival average 11.0–13.1 km/s.
- Impacts concentrate near the equator, with 24% fewer at the poles.
- Arrival times are nearly symmetrically split between local morning and evening, peaking around 6 AM/PM local time.
- A small fraction of ejecta remains in Earth co-orbital orbits, possibly feeding the near-Earth object (NEO) population.
- Objects such as Kamo’oalewa and 2024 PT5 may be examples of lunar fragments in quasi-satellite orbits.
- Understanding lunar ejecta dynamics helps reconstruct Earth’s impact history and assess meteoroid hazards.
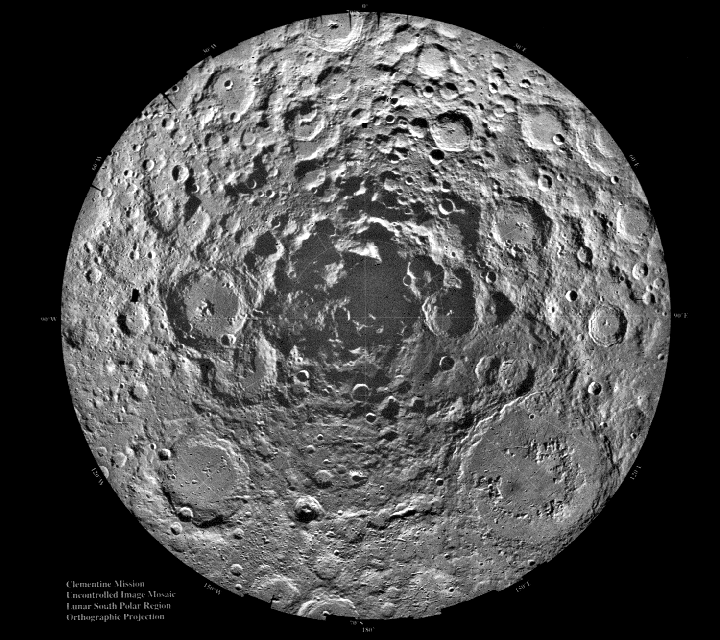
Introduction
The lunar surface is a time capsule of Solar System history. Without an atmosphere or active plate tectonics, impact craters remain preserved for billions of years, recording the intensity of bombardment during events like the Late Heavy Bombardment approximately 4 billion years ago. Each large impact excavates and ejects material, some of which attains velocities above the Moon’s escape velocity (2.38 km/s) and embarks on trajectories through cislunar space. Understanding how much of this debris reaches Earth informs our knowledge of planetary evolution, meteoroid fluxes, and even the delivery of volatile or organic materials to our planet.
Early work by Gault (1983) estimated that only about 0.5% of lunar ejecta strikes Earth, with an average accretion rate of 10–100 million grams per year. Later studies improved modeling fidelity but often treated geocentric and heliocentric phases separately. The recent study by Castro-Cisneros, Malhotra, and Rosengren integrates both phases continuously, uses a realistic ejecta velocity distribution, and extends simulations to 100,000 years, providing the most comprehensive estimate to date of the lunar ejecta flux to Earth.
Simulation Methods
The research team employed the REBOUND N-body code with the high-accuracy IAS15 integrator to simulate the trajectories of 6,000 test particles representing lunar ejecta fragments. Key aspects of their methodology include:
Parameter | Description |
---|---|
Simulation duration | 100,000 years |
Number of test particles | 6,000 |
Ejection velocity distribution | Physically motivated, tens of m-sized fragments |
Launch locations | Various lunar latitudes/longitudes (leading & trailing hemispheres) |
Gravitational bodies included | Sun, Earth, Moon, and all major planets |
Data recording interval | Every 5 years |
By modeling all gravitational influences simultaneously and using realistic velocity distributions from large cratering events, the team overcame limitations of prior two-phase studies.
Results
The simulation yielded several key findings:
Collision Probability and Timing
The total fraction of ejecta colliding with Earth over 100,000 years is 22.6%. The collision rate C(t)C(t) follows a power-law decay, C(t)∝t0.315C(t)\propto t^{0.315}, indicating most impacts occur early. Indeed, half of all collisions happen within the first 10,000 years after ejection arXiv.
Launch Hemisphere Dependence
Material launched from the Moon’s trailing hemisphere (the side opposite the direction of orbital motion) exhibits the highest Earth-collision probability, while fragments from the leading hemisphere show the lowest probability.
Launch Hemisphere | Earth Collision Probability (%) |
---|---|
Trailing | 28.4 |
Equatorial | 21.7 |
Polar | 18.0 |
Leading | 15.2 |
Impact Velocities and Geographic Distribution
Upon arrival, lunar ejecta strikes Earth at velocities between 11.0 km/s and 13.1 km/s. Impacts preferentially occur near the equator, with a 24% decrease in frequency toward the poles. The timing of impacts is nearly symmetric between local morning and evening, peaking around 6 AM/PM local time arXiv.
Implications for Planetary Science
Understanding the lunar ejecta flux has multiple implications:
-
Reconstructing Earth’s Impact History
Lunar craters serve as a proxy for Earth’s early bombardment record. By quantifying how much lunar debris returns to Earth, scientists can better correlate lunar crater ages with terrestrial impact deposits, improving timelines of events that may have influenced geological and biological evolution. -
Contribution to Near-Earth Objects (NEOs)
A minor fraction of lunar ejecta remains in co-orbital orbits for extended periods. Objects such as Kamo’oalewa (2016 HO3) and 2024 PT5 exhibit spectral signatures matching lunar material, suggesting a lunar origin. These fragments represent a hitherto underappreciated source of small NEOs. -
Meteoroid Hazard Assessment
Impact velocities of 11–13 km/s pose significant energy upon collision. Knowing the frequency and velocity distribution of lunar ejecta helps refine risk models for both Earth and spacecraft in cislunar space. -
Sample Return Opportunities
Quasi-satellite lunar fragments offer accessible targets for missions seeking pristine lunar material without landing on the Moon. Their study could reveal new information about lunar geology and impact processes.
Future Research Directions
- Oblique Impact Modeling: Incorporate non-vertical impacts to assess how lower-angle ejections alter Earth-bound flux.
- Ancient Orbital Configurations: Simulate when the Moon was closer to Earth and bombardment rates were higher, to estimate historical ejecta transfer.
- Size Distribution Effects: Extend models to different fragment sizes, from dust to boulder scale, to understand how size influences transfer efficiency.
- Spectral Surveys: Identify more lunar-origin NEO candidates via spectral matching, expanding the sample of known lunar fragments in Earth orbit.
- Sample Missions: Plan missions to quasi-satellites like Kamo’oalewa for direct sampling of lunar ejecta.
This study fundamentally revises our understanding of how much lunar material makes its way back to Earth. By demonstrating that nearly one in four ejecta fragments eventually collide with our planet—and that half do so within 10,000 years—it highlights a dynamic exchange that has shaped both lunar and terrestrial surfaces. The equatorial bias, velocity distribution, and launch-hemisphere dependence provide new parameters for modeling impact fluxes and assessing hazards. Moreover, the identification of potential lunar fragments among NEOs opens exciting avenues for future exploration and sample return.
Facts
- The Moon’s escape velocity is only 2.38 km/s, compared to Earth’s 11.2 km/s.
- Some lunar ejecta fragments spend tens of thousands of years orbiting the Sun before hitting Earth.
- Meteorites found on Earth that originate from the Moon are called “lunar meteorites.”
- The largest known lunar crater, South Pole–Aitken Basin, is over 2,000 km across.
- Earth receives hundreds of tons of meteoritic material daily, but only a small fraction comes from the Moon.
References
- Castro-Cisneros, J. D., Malhotra, R., & Rosengren, A. J. (2025). Lunar impact ejecta flux on the Earth. arXiv:2504.15502. arXiv
- Investigation of lunar ejecta dynamics: particles reaching the near Earth. A&A. A&A
- Gladman, B., et al. (1995). The dynamical evolution of lunar impact ejecta. Icarus, 118, 302–321. ADS
- Gault, D. E. (1983). Accretion rate of lunar ejecta onto Earth. JGR, 88, A31–A35. A&A
- NASA Lunar Reconnaissance Orbiter observations of meteoroid impacts. NASA Release 16-33. NASA
- University of Arizona study on lunar fragment Kamo’oalewa. EurekAlert! EurekAlert!
- Sharkey, J., et al. (2021). Spectral analysis of Kamo’oalewa. AJ. PubMed
- Mitchell, E. K., et al. (2024). Lunar ejecta origin of near-Earth asteroid Kamo’oalewa. Commun. Earth Environ. PubMed
- Reuters (2024). Meteorite impacts drive Moon’s tenuous atmosphere. Reuters
- Wired (2012). New NASA video depicts the Moon’s fiery history. WIRED