Earth 2.0: How ESA’s PLATO Mission Could Redefine Exoplanet Science
The European Space Agency’s PLATO mission will launch in 2026. This mission wants to change how we find Earth-like planets outside our Solar System. It will look at up to one million stars. Scientists will watch for small dips in a star’s brightness. This is called a planetary transit. It happens when a planet passes in front of a star. PLATO will use advanced technology. It will also use many telescopes together. This means it can find Earth-like planets more accurately than before. The mission might find planets where living things could exist. It could even find signs of life. This will help us understand the universe better. We might even find a planet just like Earth. We call this idea “Earth 2.0.”
Summary
- PLATO’s mission could confirm thousands of rocky exoplanets in habitable zones.
- Its multi-telescope system includes 26 cameras designed for precision.
- Focused on G-type stars, it overcomes previous detection limitations of Earth-like planets.
- PLATO’s stellar variability program reduces noise interference.
- Combines space-based observations with ground-based follow-up studies.
- Supported by the ESA’s exoplanet missions, including CHEOPS and ARIEL.
- Works alongside NASA’s James Webb Space Telescope and future ground-based observatories.
- Utilizes solar variability models based on NASA’s Solar Dynamics Observatory.
- Expected to detect Earth-sized planets with orbital periods of 200-500 days.
- Advances in detecting biosignatures (oxygen, methane, water vapor) are anticipated.
- The mission leverages interdisciplinary approaches across astronomy, physics, and data science.
- Will address current limitations in detecting smaller signals from Earth-like planets.
- Complements the capabilities of other exoplanet discovery tools, such as radial velocity techniques.
- Could enable scientists to differentiate between “potentially habitable” and “habitable.”
- Groundbreaking in its ability to identify truly “Earth 2.0” candidates.
Introduction to Exoplanet Science
Exoplanets are planets that exist outside our solar system. They have fascinated scientists ever since they confirmed the first one in 1992. By 2024, scientists have found over 5,700 exoplanets. These exoplanets are in 4,300 different star systems. Most of them are either gas giants or Super-Earths. Gas giants are large planets made mostly of gas, and Super-Earths are planets larger than Earth but smaller than gas giants.
Finding planets like Earth has been difficult. Scientists look for rocky planets that have similar mass and size as Earth. They want to find these planets in the habitable zones of stars like our Sun. The habitable zone is the area around a star where conditions might be right for life. But locating these true Earth analogs has been hard.
This limitation exists because of current telescope technologies. These technologies struggle to detect smaller planets. It is also hard for them to find planets with longer orbital periods. Orbital period is the time a planet takes to travel around a star. The European Space Agency has a mission named PLATO. It promises to overcome these challenges. PLATO will have advanced photometric precision. Photometric precision is the ability to measure light very accurately. PLATO aims to change the field of exoplanet science.
PLATO: A New Era in Exoplanet Detection
PLATO (PLAnetary Transits and Oscillations of stars), scheduled for launch in 2026, is a next-generation space observatory. Unlike its predecessors, PLATO uses an innovative multi-telescope approach, housing 26 cameras capable of detecting minute dimming caused by transiting planets. This configuration enables the detection of rocky, Earth-like exoplanets even if only a single transit event occurs.
Table 1: Key Features of PLATO Mission
Feature | Details |
---|---|
Launch Year | 2026 |
Telescope Configuration | 26 cameras (24 normal, 2 fast) |
Focus Area | G-type (Sun-like) stars |
Detection Method | Transit Photometry |
Observation Strategy | Continuous 2-year monitoring of each star |
The focus of the PLATO mission is to detect and characterize Earth-sized planets orbiting within the habitable zones of Sun-like stars. It achieves this by combining high-precision photometry, stellar variability analysis, and ground-based follow-up campaigns.
Why Focus on Sun-like Stars?
Sun-like (G-type) stars offer the most promising conditions for habitability. These stars provide stable energy output and fall within a temperature range conducive to liquid water, a fundamental ingredient for life.
The Science Behind Transit Photometry
Transit photometry is a method used to study stars far away. It measures the light from these stars over time. Scientists look for regular dimming in the light. This dimming happens when a planet moves in front of the star. Astronomers have found 74.5% of all known exoplanets using this technique. PLATO is a tool that improves this method. It is more sensitive and can notice very tiny changes in light. PLATO can detect changes as small as 0.0084%. This is the same as how much the Earth dims the Sun when it passes in front of it.
However, transit photometry faces challenges. Noise from stellar variability is one challenge. Another challenge is limitations of the instruments. PLATO addresses these issues. Solar variability models help with the problem. These models describe changes in the sun’s brightness. PLATO also uses advanced algorithms to reduce noise. Algorithms are step-by-step procedures for calculations.
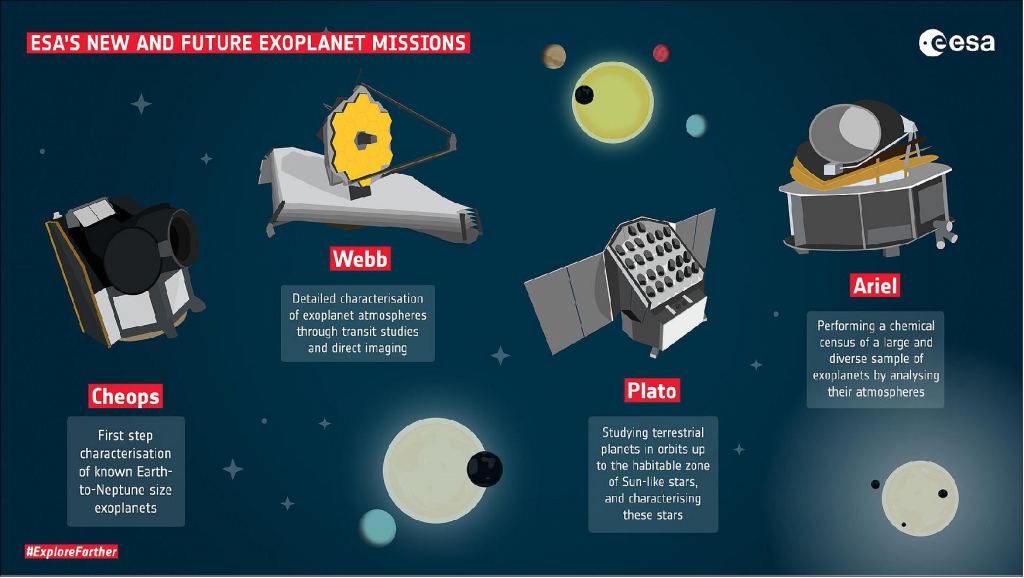
Modeling PLATO’s Potential
To evaluate how well PLATO performs, scientists used solar data. This data came from NASA’s Helioseismic and Magnetic Imager (HMI). Scientists added Earth-like transit signals into the data. A transit signal is a dip in a star’s brightness that indicates a planet is passing in front of the star. By doing this, they simulated observations of stars similar to our Sun under different conditions.
Their findings indicate that PLATO can reliably detect Earth-sized planets even around faint stars. Moreover, its advanced algorithms ensure accurate size measurements of these planets, a crucial factor in determining their potential habitability.
Table 2: Comparison of Exoplanet Detection Missions
Mission | Focus | Key Achievements |
---|---|---|
Kepler | Broad survey of exoplanets | Discovered over 2,600 planets |
CHEOPS | Characterization | Refined size/mass measurements |
PLATO | Earth-like planets | Detects single-transit events, habitable zones |
JWST | Atmospheric analysis | Detects biosignatures |
The Broader Implications
PLATO works alongside other future space missions. One example is NASA’s James Webb Space Telescope (JWST). Another is ESA’s ARIEL. PLATO’s main job is to find exoplanets. Exoplanets are planets outside our solar system. JWST helps by studying the atmospheres of these planets. They work together. This partnership helps us learn more about exoplanets that might support life.
These missions might soon help scientists find clear signs of life. These signs include oxygen, methane, and water vapor. Scientists will look for these on planets outside our solar system, called exoplanets. The missions will also study the surface conditions on these planets. They will examine how the atmospheres work. This will help scientists decide if these planets could support life.
The implications of PLATO’s discoveries extend beyond science, potentially shaping humanity’s search for Earth 2.0. By identifying true Earth analogs, PLATO could lay the groundwork for future interstellar missions, furthering our understanding of life beyond Earth.
Facts About Exoplanet Exploration
- The term “exoplanet” was first coined in the late 20th century.
- Most exoplanets are discovered using indirect methods like transit photometry or radial velocity.
- The closest known exoplanet, Proxima Centauri b, lies just 4.24 light-years away.
References
- Recent Study
- Andreas F. Krenn
- Space Research Institute at the Austrian Academy of Sciences
- Observatoire Astronomique de l’Université de Genève, Aix Marseille University
- Columbia Astrophysics Laboratory
- Leibniz Institute for Astrophysics Potsdam
- Institute of Astronomy at KU Leuven
- National Center for Atmospheric Research
- Kanzelhöhe Observatory for Solar and Environmental Research
- Astronomy & Astrophysics
- ESA’s CHaracterising ExOPlanets Satellite
- https://www.esa.int/Science_Exploration/Space_Science/Plato
- PLAnetary Transits and Oscillations of stars (PLATO)
- James Webb Space Telescope (JWST)
- Atmospheric Remote-sensing Infrared Exoplanet Large-survey
- Nancy Grace Roman Space Telescope
- Astronomy & Astrophysics