Why Scientists Say the Universe is 13.8 Billion Years Old
Key Takeaways
- The Universe is estimated to be 13.8 billion years old, based on measurements of the cosmic microwave background, the expansion rate of the Universe, and the age of the oldest known stars.
- Two primary methods for determining the Universe’s age involve dating the oldest objects and applying general relativity to the expanding Universe.
- The Hubble tension, a discrepancy between different measurements of the Universe’s expansion rate, poses a challenge to the current age estimate but does not significantly alter it.
- Cosmic inflation, a rapid expansion before the hot Big Bang, suggests that the Universe could be older than 13.8 billion years, but this remains speculative.
- The age of the Universe is a crucial aspect of modern cosmology, providing insights into the origins and ultimate fate of the cosmos.
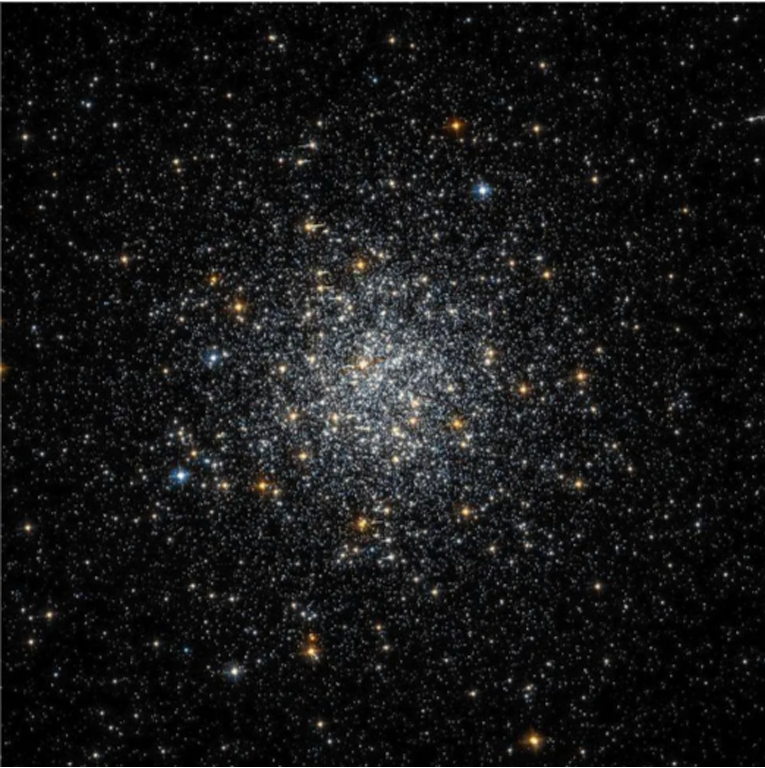
The Hot Big Bang Theory: The Universe’s Beginning?
The theory of the hot Big Bang suggests that the Universe had a definitive beginning, often described as “a day without a yesterday.” This idea, once controversial and mind-boggling, is now a cornerstone of modern cosmology. The concept of a beginning to the Universe aligns with some religious texts, causing skepticism among certain circles. However, from a scientific perspective, the hot Big Bang is not the absolute start of the Universe but rather the aftermath of a preceding epoch, possibly cosmic inflation.
Despite this nuance, when asked about the age of the Universe, cosmologists and astrophysicists consistently respond with “13.8 billion years.” This figure has been reached through various methods, including the analysis of the CMB and the study of distant galaxies and star clusters. But where do we start counting the Universe’s age, and what are the implications of this starting point?
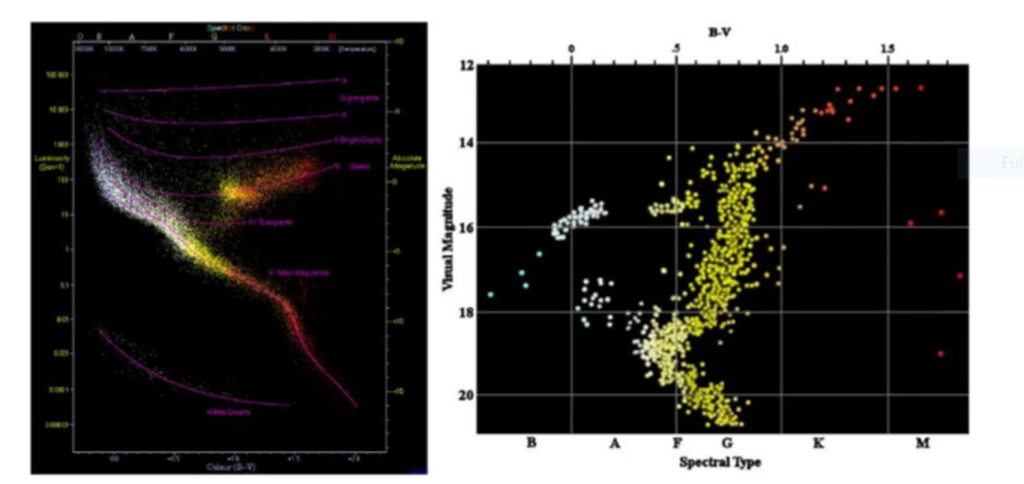
Measuring the Universe’s Age: Two Main Methods
There are two primary approaches to determining the age of the Universe:
- Dating the Oldest Objects: By measuring the age of the oldest stars or star clusters, we can establish a lower bound for the Universe’s age.
- Cosmic Expansion and General Relativity: By applying our understanding of general relativity and the known components of the Universe, we can calculate the time elapsed since the hot Big Bang.

In the middle panel, space could have had any curvature. Inflation made the space expand so much that we can’t see any curvature today. This solves the flatness problem.
In the bottom panel, high-energy relics existed before. Inflation pushed these relics away. This solves the high-energy relic problem.
These examples show how inflation solves three major puzzles. The Big Bang alone cannot explain these puzzles. (Credit: E. Siegel/Beyond the Galaxy)
Method 1: Dating the Oldest Objects
As cosmology evolved from astronomy and physics, one of the first reliable methods for estimating the age of the Universe involved studying the oldest stars and star clusters. Globular clusters, in particular, are dense groups of stars that formed early in the Universe’s history. These clusters are invaluable for estimating the age of the Universe.
Globular clusters contain stars of varying masses, colors, and lifespans. The most massive and brightest stars exhaust their nuclear fuel quickly, leaving behind only cooler, dimmer stars. By studying these remaining stars and the absence of their massive counterparts, scientists can estimate the age of the cluster, which often exceeds 12 billion years. This method provides a lower bound for the Universe’s age, confirming that it must be at least as old as the oldest stars, around 12.5 to 13 billion years.
Method 2: Cosmic Expansion and General Relativity
The second method involves applying general relativity to the expanding Universe. The Friedmann equations, derived from Einstein’s general relativity, describe how the Universe expands over time. By inputting data such as the current expansion rate (known as the Hubble constant) and the composition of the Universe, scientists can calculate how long it has been expanding since the hot Big Bang.
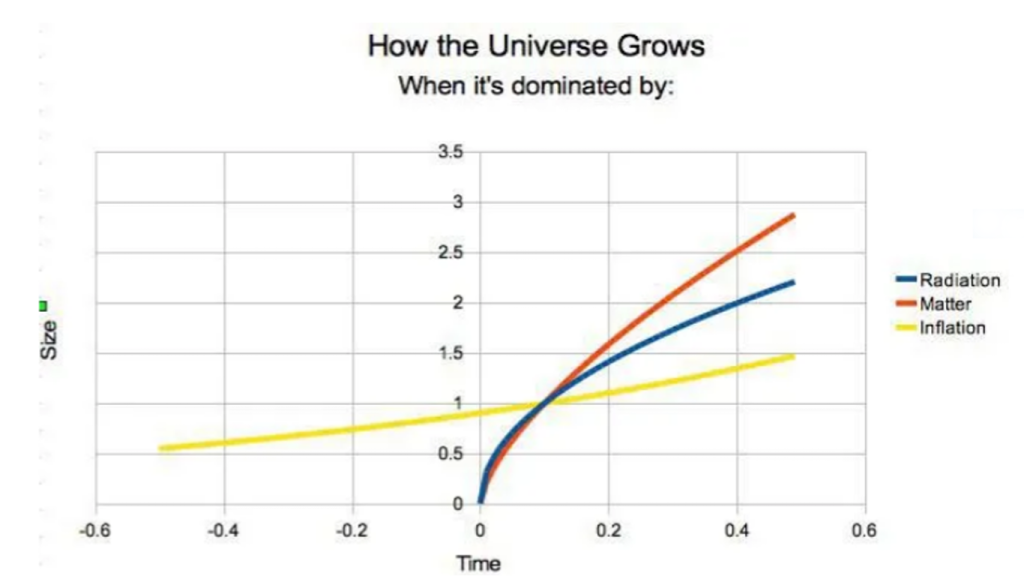
In an inflationary scenario, shown in yellow, we never reach a singularity. A singularity is a point where space is infinitely small. Instead, space just becomes very small in the past while time keeps going backward forever.
The last tiny fraction of a second, from the end of inflation, leaves its mark on our observable Universe today.
At the start of the hot Big Bang, the size of the now-observable Universe could not have been smaller than about 1 cubic meter in volume.
(Credit: E. Siegel)
The most accurate data for this calculation comes from the CMB, the remnant radiation from the Big Bang, and large-scale galaxy clustering. The Universe’s composition is primarily:
- 68% dark energy
- 27% dark matter
- 4.9% normal matter
- 0.1% neutrinos
- 0.01% photons
Given these proportions and an expansion rate of 67 km/s/Mpc, the calculations yield an age of approximately 13.8 billion years. However, this conclusion is not without contention.
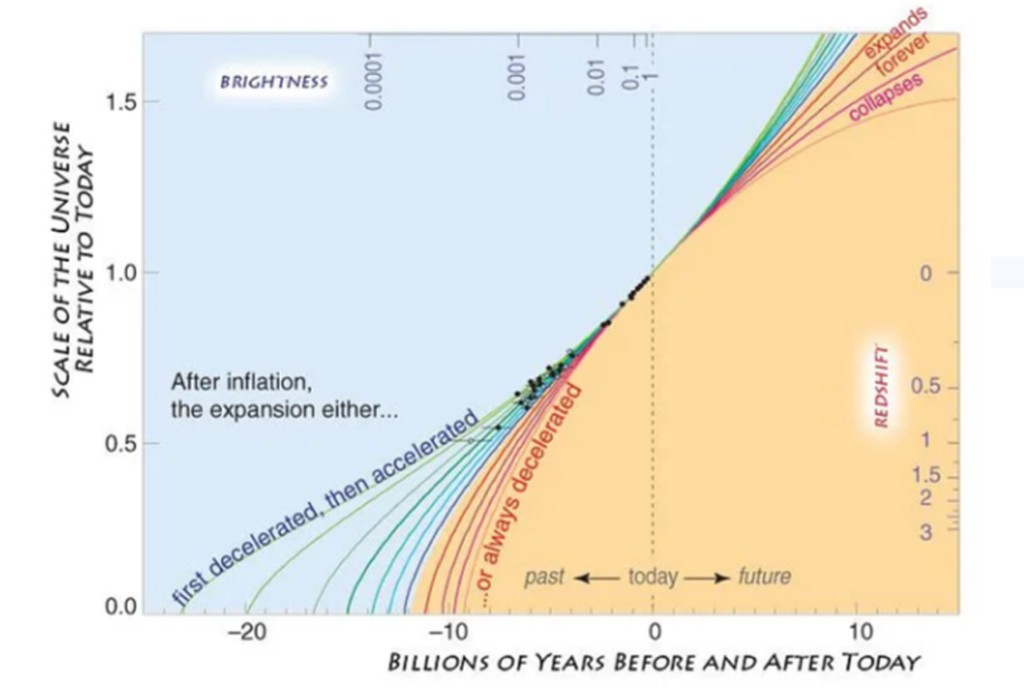
In the late 1990s, data from exploding stars called supernovae showed something surprising. The data suggested that the Universe has a lot of dark energy, not just matter and radiation. This was a new discovery. (Credit: Saul Perlmutter/UC Berkeley)
The Hubble Tension: A Challenge to the Age of the Universe?
One of the most significant challenges to the current estimate of the Universe’s age is the so-called Hubble tension. This discrepancy arises because different methods of measuring the Hubble constant (the Universe’s expansion rate) yield slightly different values. Early Universe measurements, like those from the CMB, suggest a rate of 67 km/s/Mpc, while late-time methods, such as the cosmic distance ladder, indicate a higher rate of around 73-74 km/s/Mpc.
If the higher rate is correct, it could imply a younger Universe, possibly around 13.6 billion years. However, the relationship between the expansion rate, dark energy, and dark matter introduces complexities. A faster expansion rate would require adjusting the proportions of dark energy and dark matter, slightly lowering the Universe’s age but not drastically altering it. Even with this adjustment, the difference is marginal, reinforcing the robustness of the 13.8 billion-year estimate.
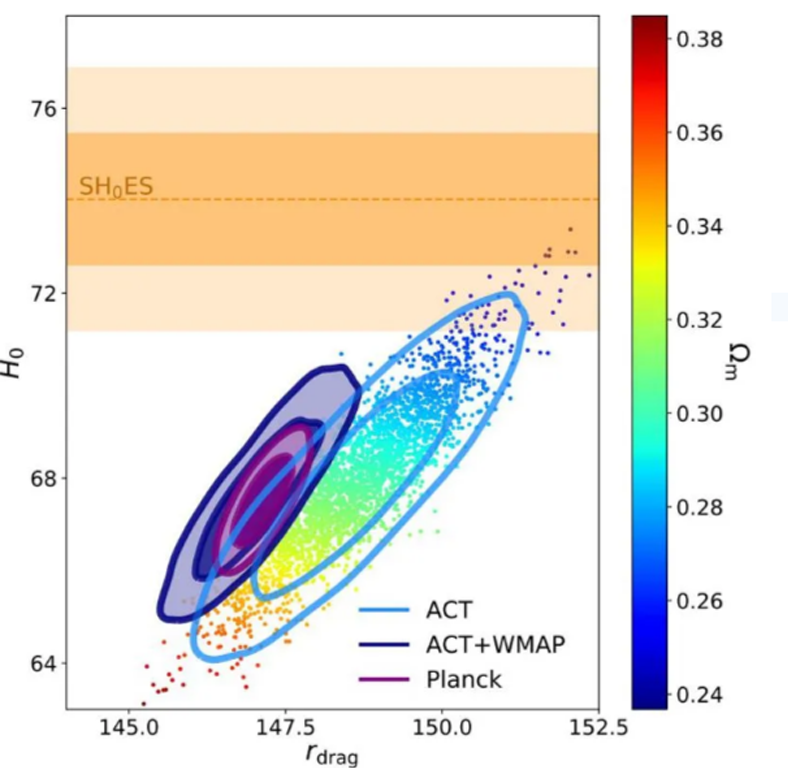
Cosmic Milestones: When Should We Start Counting?
Another intriguing question is when to start counting the Universe’s age. The CMB, which we observe today, was emitted 380,000 years after the Big Bang when the Universe cooled enough for neutral atoms to form. But should we start counting from this point, or should we go back further to earlier milestones?
Several significant events occurred before the CMB was emitted, such as Big Bang nucleosynthesis (which took place just minutes after the Big Bang) and the formation of the cosmic neutrino background, which imprinted itself when the Universe was just one second old. These events suggest that counting should start even earlier than the CMB, although the difference is negligible in the context of 13.8 billion years.
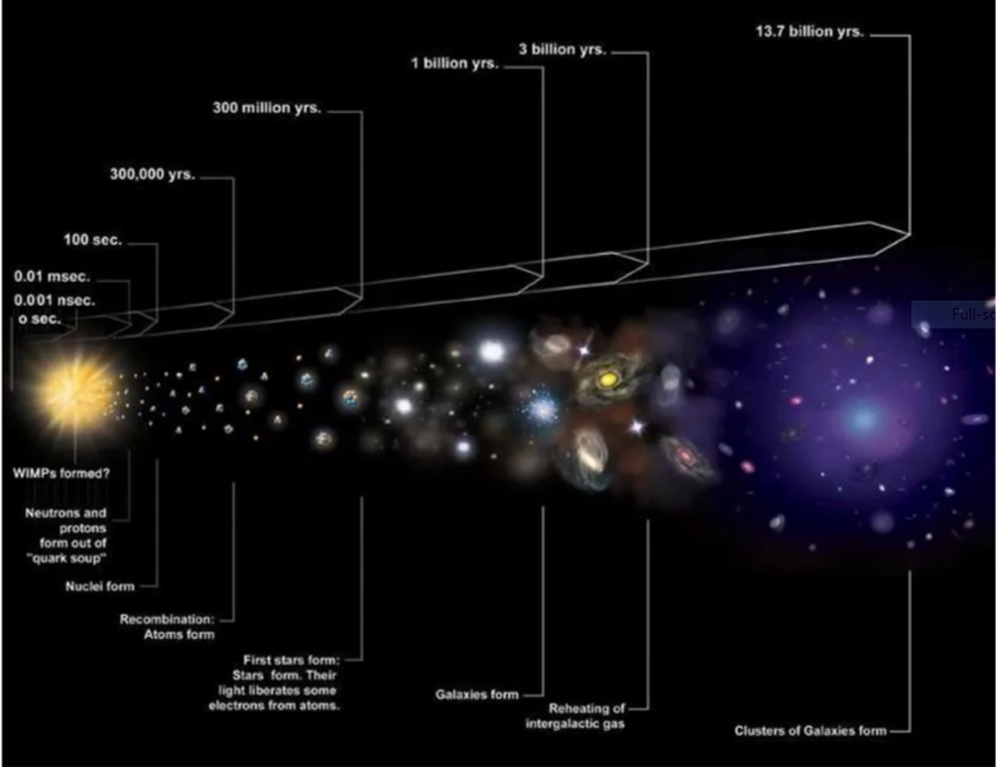
The Role of Cosmic Inflation: What Came Before the Big Bang?
One of the most fascinating aspects of modern cosmology is the realization that the hot Big Bang may not have been the true beginning. Before the Big Bang, the Universe likely underwent a period of cosmic inflation, a rapid expansion driven by a high-energy state. This inflation smoothed out any irregularities and set the stage for the Big Bang.
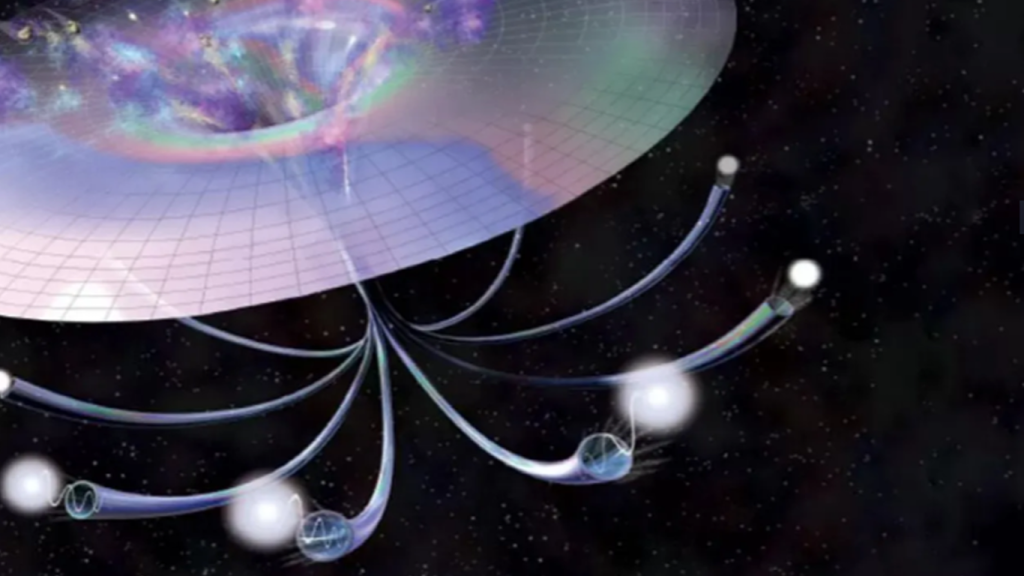
If cosmic inflation preceded the Big Bang, then the Universe’s true age could be even greater than 13.8 billion years. However, the duration of inflation is uncertain, and it could have lasted for an extraordinarily brief time. Thus, the age of the Universe is conventionally measured from the start of the hot Big Bang, as this is the earliest Era we can confidently describe using known physics.
The Universe’s age of 13.8 billion years is a well-supported estimate based on multiple lines of evidence. From the oldest stars to the cosmic microwave background and the expansion of space itself, all indicators converge on this figure. While there are challenges and differences, such as the Hubble tension and the role of cosmic inflation, the fundamental conclusion remains robust.
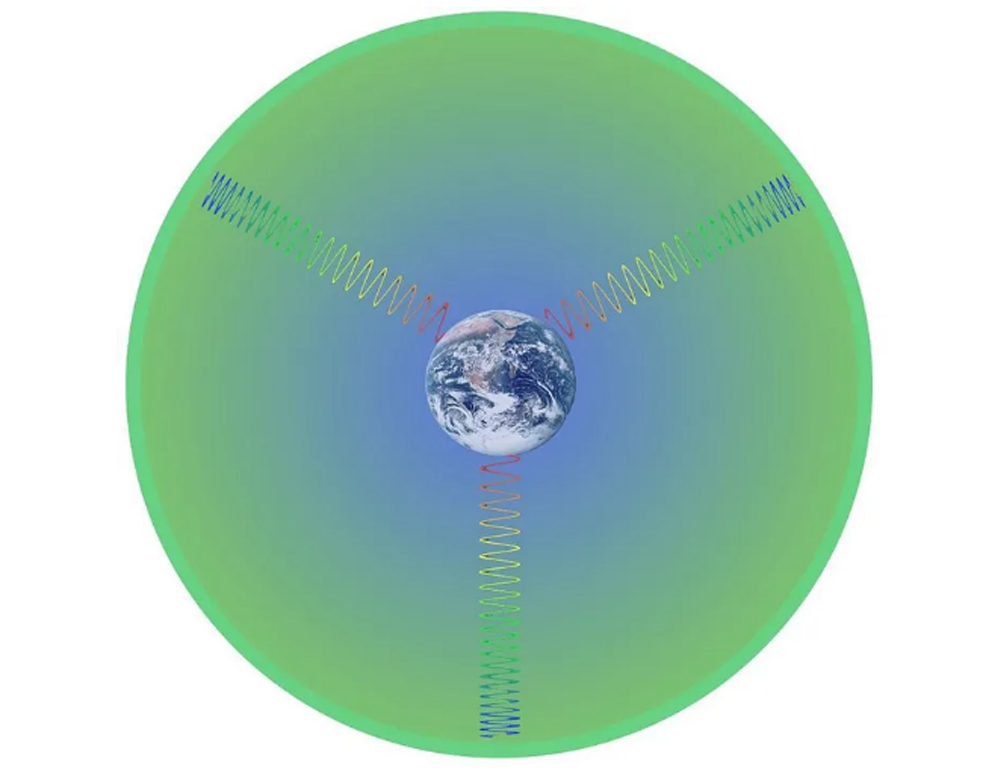
Whether the Universe’s true beginning was the hot Big Bang or an earlier inflationary phase, the age of 13.8 billion years remains a cornerstone of modern cosmology. This understanding not only informs us about the past but also provides a foundation for exploring the Universe’s future and the ultimate fate of all that exists.