Even Stars Can Get the Hiccups: Exploring Cosmic Anomalies and Their Causes
The concept of “stellar hiccups” reveals a fascinating phase in the lives of massive stars, where rapid core expansions and contractions can precede supernova explosions. This newly observed phenomenon, known as “pulsational pair-instability,” enhances our understanding of stellar evolution and the cosmic processes that shape the universe.
Summary
- Stellar hiccups are rare, observable pre-supernova phases in stars with masses ranging between 60-150 times that of the Sun.
- The phenomenon is caused by pulsational pair-instability (PPI), where the stellar core rapidly contracts and expands under extreme temperatures.
- Massive stars nearing the end of their lifespans eject shells of material during these “hiccup” events, creating bursts of energy visible from Earth.
- These “hiccups” help scientists understand how massive stars shed mass and transition to the supernova stage.
- The discovery of SN2020acct in the NGC2981 galaxy provided the first-ever observation of this phenomenon.
- The core mechanism involves material ejection due to unstable thermonuclear reactions in massive stars, followed by collisions between ejected shells of gas.
- This process was theorized for decades but remained unobserved due to its rarity and faintness.
- Observing hiccups can aid in predicting supernova occurrences and understanding element distribution in the universe.
- The remnants of these massive explosions create neutron stars or black holes, depending on the progenitor’s mass.
- The study also sheds light on the role of supernovae in spreading heavy elements critical for forming planets and life.
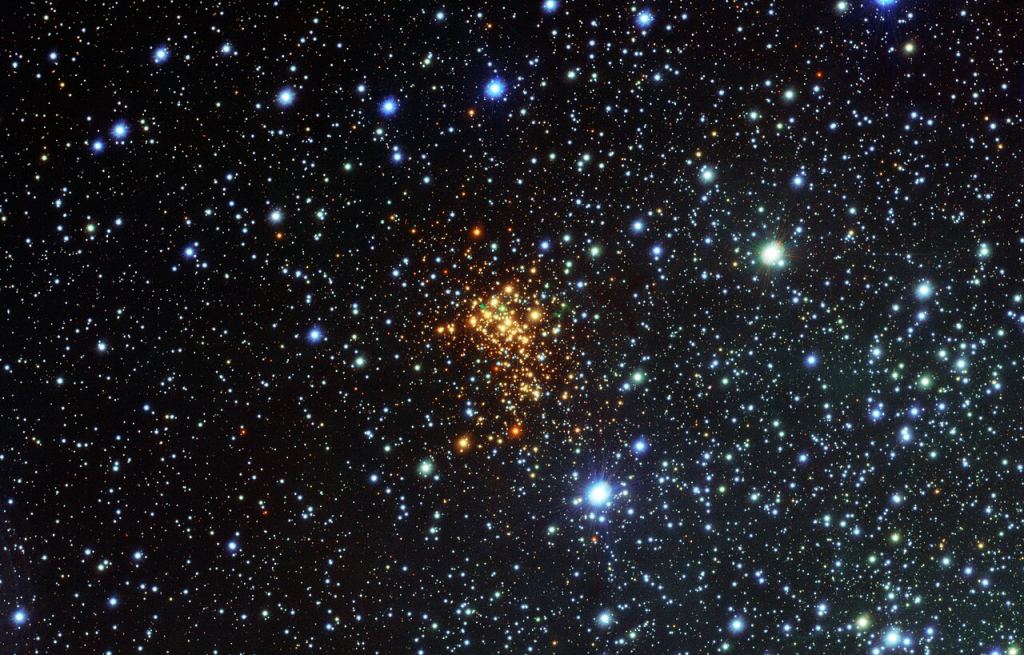
However, we can’t see this cluster clearly because gas and dust block most of its visible light from reaching Earth. Recently, astronomers found something unexpected while studying images of Westerlund 1. These images are from a new survey of the southern skies. They discovered clouds of glowing hydrogen gas around one of the stars in the cluster. This star is called W26. W26 is a red supergiant and might be the biggest star known.
Glowing clouds around massive stars are very rare. They are even rarer around a red supergiant. In fact, this is the first ionised nebula found around such a star. An ionised nebula is a glowing cloud of gas that usually surrounds stars. W26 is too cool to make the gas glow by itself. The astronomers think that the gas glows due to radiation from somewhere else. The source might be hot blue stars elsewhere in the cluster or a much hotter companion star to W26.
W26 will eventually explode as a supernova. A supernova is a powerful explosion that happens when a star dies. The nebula around W26 is similar to the one that surrounded SN1987A. SN1987A is the remains of a star that became a supernova in 1987. It was the closest supernova to Earth observed since 1604. This gave astronomers a chance to learn more about these explosions.
By studying objects like the new nebula around W26, astronomers can understand how massive stars lose mass before exploding. Understanding these processes helps scientists learn more about the life and death of stars.
This picture is part of a detailed survey of a large part of the Milky Way. The survey is called VPHAS+ and uses the VST’s power to find new objects like young stars and planetary nebulae. A planetary nebula is a glowing shell of gas and dust around an old star. A recent picture of the Prawn Nebula also came from this survey.
Cosmic Context of Stellar Hiccups
Stars are colossal nuclear furnaces, responsible for producing and dispersing heavy elements essential for the formation of planets and life. Among these stars, massive ones often live dramatically short lives, culminating in supernova explosions that distribute their materials into space. However, before the grand finale of a supernova, some stars exhibit unique “hiccups” due to a rare process called pulsational pair-instability (PPI).
What Are Stellar Hiccups?
PPI causes the cores of massive stars to rapidly expand and contract, ejecting shells of material in the process. These hiccups are short-lived, occurring just years, or even days, before a supernova.
In December 2020, astronomers discovered one such hiccup in the galaxy NGC2981, marking the first observation of this fascinating event.
The Science Behind Pulsational Pair-Instability
The term pulsational pair-instability refers to a rare phenomenon where conditions in a star’s core destabilize due to:
- Extreme Heat: Stars exceeding 60 times the Sun’s mass reach temperatures high enough to produce electron-positron pairs, reducing radiation pressure.
- Core Collapse: Reduced pressure causes the core to collapse under gravity.
- Rapid Expansion: Nuclear reactions reignite, causing the core to expand and eject material in violent bursts.
How PPI Affects Stellar Evolution
Each hiccup expels part of the star’s mass, lowering its overall size and altering its eventual fate. Over time, the remaining core becomes unstable enough to collapse into either a neutron star or a black hole.
Observed Phenomenon: The Case of SN2020acct
The Fred Lawrence Whipple Observatory detected SN2020acct, initially classified as a supernova. However, astronomers later discovered that the light emitted was not a supernova but the result of material shells colliding near the star.
Observation Timeline | Key Events |
---|---|
December 2020 | SN2020acct discovered in NGC2981 |
February 2021 | Unusual light reappeared in the same region |
Detailed Analysis | Confirmed “hiccups” as the cause |
Why Are Stellar Hiccups Important?
Stellar hiccups provide insights into the processes that precede supernovae, which are critical for understanding:
- Elemental Formation: The heavy elements necessary for life are created during these events.
- Massive Star Evolution: PPI events help explain how massive stars lose mass before exploding.
- Supernova Prediction: Observing hiccups can refine supernova timelines, aiding astronomical studies.
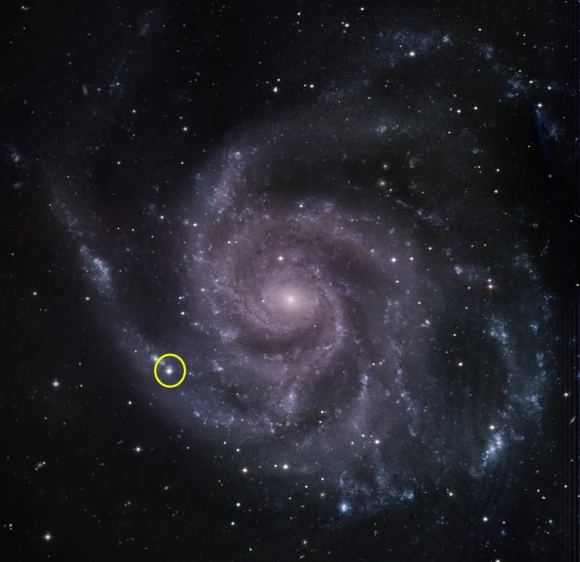
Supernovae: The Aftermath of Stellar Hiccups
Supernovae are categorized into two primary types:
Supernova Type | Key Features |
---|---|
Type I | Occurs in binary star systems; involves the accumulation of matter on a white dwarf. |
Type II | Marks the death of a massive star; involves core collapse and violent expulsion of outer layers. |
Facts About Stellar Hiccups
- Stellar hiccups are believed to occur in stars 60-150 times the mass of the Sun.
- The phenomenon was only theorized until its first observation in 2020.
- Hiccups can lead to repetitive light bursts from stars before they die.
- The Pinwheel Galaxy (Messier 101) recently hosted one of the brightest supernova events related to stellar hiccups.
Applications and Future Research
Astronomers aim to leverage telescopic advancements to:
- Detect more stars exhibiting hiccups.
- Study their frequency and duration.
- Develop models predicting supernova timings.
Stellar hiccups provide a rare glimpse into the chaotic lives of massive stars nearing their end. Observing these events enhances our understanding of supernovae, the creation of heavy elements, and the intricate processes that govern our universe.
The discovery of SN2020acct marked a pivotal moment in astronomy, highlighting the importance of continued research into cosmic anomalies. As technology advances, astronomers hope to unlock more secrets of the universe, expanding humanity’s understanding of the cosmos.
References
- Hiccuping Stars Caught in Action – Queen’s University Belfast
- Fred Lawrence Whipple Observatory – Center for Astrophysics