Solar Eclipse vs. Lunar Eclipse: Understanding the Differences
Solar eclipses occur when the Moon passes between the Earth and the Sun, temporarily blocking the Sun’s light, whereas lunar eclipses happen when the Earth comes between the Sun and the Moon, casting its shadow on the Moon. Both phenomena are breathtaking and scientifically valuable, offering unique insights into our celestial mechanics.
Summary
- Solar Eclipses: Occur during a new moon when the Moon positions itself between the Sun and Earth.
- Lunar Eclipses: Happen during a full moon when Earth interposes itself between the Sun and the Moon.
- Orbital Dynamics: The Moon’s orbit is tilted about 5 degrees relative to Earth’s orbit, which is why perfect alignments are rare.
- Observation Safety: Solar eclipses require special eye protection, whereas lunar eclipses are safe to view with the naked eye.
- Types of Solar Eclipses: Include partial, annular (ring of fire), and total eclipses, each with distinct visual characteristics.
- Types of Lunar Eclipses: Range from penumbral and partial to total (blood moon), with varying levels of darkness and color change.
- Frequency: Eclipses occur in eclipse seasons lasting 31 to 37 days, with multiple events possible within each season.
- Cultural Significance: Historically, eclipses have been interpreted as omens, divine messages, and pivotal events in various cultures.
- Scientific Impact: They provide opportunities to study the Sun’s corona, the Earth’s atmosphere, and the mechanics of celestial movements.
- Upcoming Events: Notable events include the total solar eclipse on Aug. 12, 2026 and the total lunar eclipse on March 13-14, 2025.
- Educational Resources: Further details can be found on NASA’s Eclipse Website, Space.com, and Eclipse Wise.
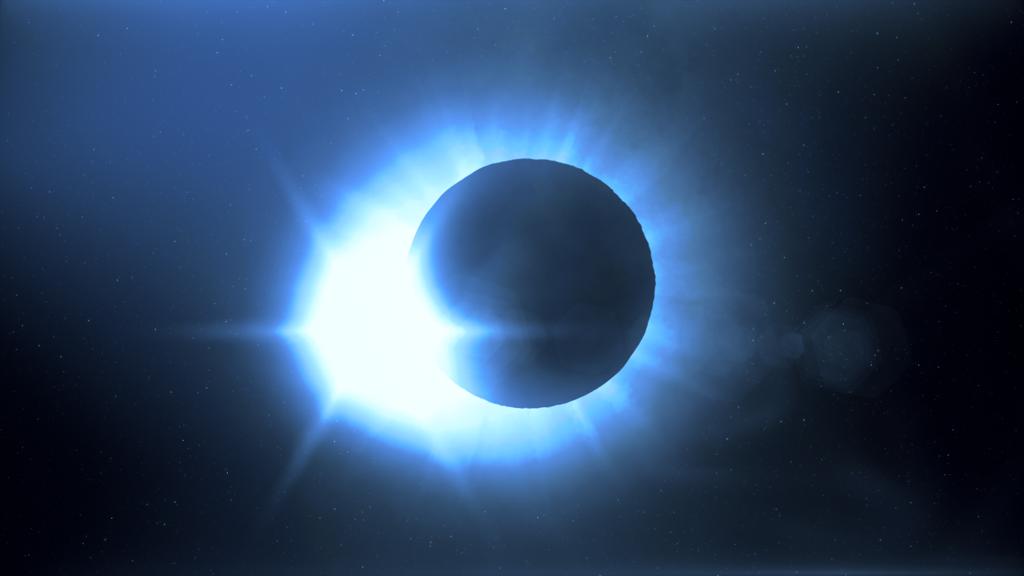
Introduction
Eclipses have grabbed people’s attention for ages, creating amazement, a desire to learn, and even worry. These special happenings, made by how the Earth, Moon, and Sun work together, are amazing to watch and also give a way to make scientific finds. Solar and lunar eclipses both use shadows and light, but how they happen, look, and feel to those watching are very different. We will go over these differences, check out the kinds of eclipses, and talk about why these events keep drawing people in around the globe.
What Causes a Solar Eclipse?
A solar eclipse occurs when the Moon moves directly between the Sun and Earth. This alignment causes the Moon to block a portion of the Sun’s light, casting a shadow on Earth. It is important to note that a solar eclipse can only take place during a new moon, when the Moon is positioned directly in front of the Sun. One might wonder how the Moon, which is roughly 400 times smaller than the Sun, can cover it completely. The answer lies in the fact that the Sun is about 400 times farther away from Earth than the Moon, making their apparent sizes nearly equal in our sky. This perfect balance leads to various types of solar eclipses:
Partial Solar Eclipse: Only part of the Sun is obscured by the Moon. Special solar filters or eclipse glasses are required to safely observe this event.
Annular Solar Eclipse (Ring of Fire): The Moon covers the center of the Sun, leaving a bright ring visible around the edges.
Total Solar Eclipse: The Moon completely covers the Sun, allowing observers in the path of totality to witness the rare sight of the solar corona—the Sun’s outer atmosphere.
For more detailed insights, please visit NASA’s Eclipse Website.
What Causes a Lunar Eclipse?
A lunar eclipse happens when Earth comes between the Sun and the Moon, causing Earth’s shadow to fall upon the lunar surface. Unlike solar eclipses, lunar eclipses occur during a full moon. Despite the regular occurrence of full moons, lunar eclipses remain relatively rare because of the Moon’s orbital tilt relative to Earth’s orbital plane around the Sun. When the alignment is just right, different phases of a lunar eclipse can occur:
Penumbral Lunar Eclipse: The Moon passes through Earth’s lighter penumbral shadow, leading to a subtle dimming that is often hard to detect.
Partial Lunar Eclipse: Only a portion of the Moon moves into the darkest part of Earth’s shadow, known as the umbra, creating noticeable shading.
Total Lunar Eclipse (Blood Moon): The entire Moon enters the umbra, and due to the Earth’s atmosphere filtering the sunlight, the Moon takes on a reddish hue—a phenomenon that has earned it the name “Blood Moon.”
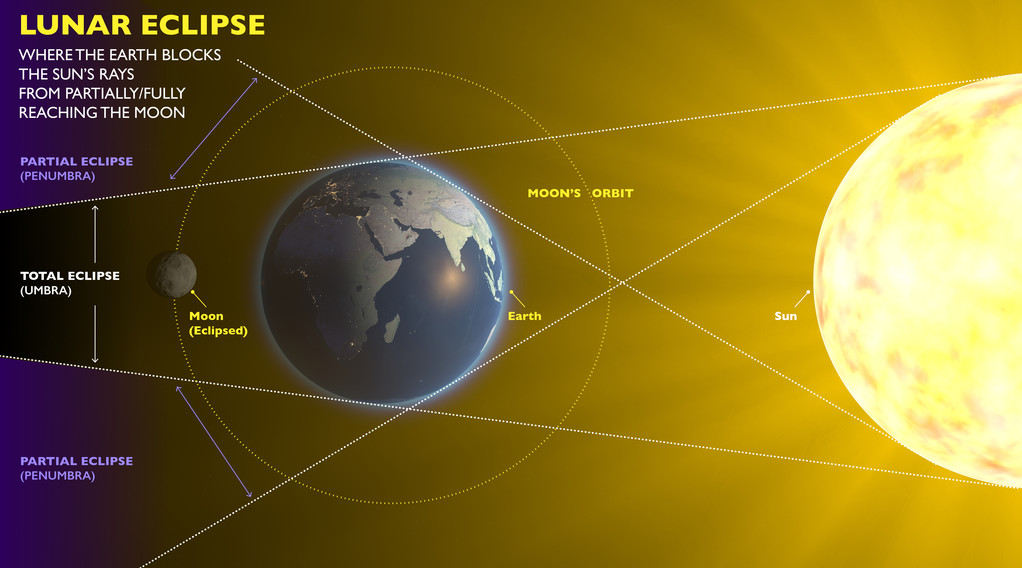
Comparative Analysis of Solar and Lunar Eclipses
Below is a table summarizing the core differences between solar and lunar eclipses:
Aspect | Solar Eclipse | Lunar Eclipse |
---|---|---|
Occurrence | New moon; Moon positioned between Sun and Earth | Full moon; Earth positioned between Sun and Moon |
Visibility | Limited to a narrow path on Earth | Visible from any location on Earth with a view of the Moon |
Observation | Requires protective eyewear to prevent eye damage | Safe to view directly with the naked eye |
Types | Total, Partial, Annular (Ring of Fire) | Penumbral, Partial, Total (Blood Moon) |
Detailed Types of Eclipses
Solar Eclipses
Solar eclipses vary based on the alignment and distance of the Moon from Earth. A partial solar eclipse is observed when only a section of the Sun is obscured. The annular solar eclipse, known as the Ring of Fire, occurs when the Moon covers the center of the Sun, leaving a luminous ring visible around the edges. Finally, the total solar eclipse completely covers the Sun, momentarily revealing the ethereal solar corona. These events are not only visually stunning but also serve as important opportunities for scientific exploration, especially in studying the Sun’s outer layers.
Lunar Eclipses
The phases of a lunar eclipse can be quite dramatic. In a penumbral lunar eclipse, the Moon experiences only a slight dimming as it passes through Earth’s penumbra. During a partial lunar eclipse, part of the Moon enters the umbra, causing a distinct darkening. When the entire Moon is engulfed by the umbra, a total lunar eclipse—often called a Blood Moon—occurs, turning the Moon a deep red. This phenomenon results from the Earth’s atmosphere bending and filtering sunlight, a process that adds a mystical quality to the event.
Eclipse Occurrence and Frequency
Eclipses occur during specific periods known as eclipse seasons, which last between 31 and 37 days. During these seasons, the alignment of the Earth, Moon, and Sun allows for the possibility of multiple eclipses—ranging from four to seven in a single season. While solar eclipses are visible only along a narrow geographic path and a total solar eclipse may occur at any given location only once every few centuries, lunar eclipses are visible from any location on Earth where the Moon is above the horizon. According to NASA, a total solar eclipse is visible somewhere on Earth roughly every 18 months, whereas a total lunar eclipse can be observed from any given location about once every 2.5 years. For updated eclipse schedules, please visit Eclipse Wise.
Upcoming Eclipses and Observation Tips
Below is a table highlighting some of the upcoming eclipses along with tips for safely observing these phenomena:
Eclipse Type | Next Occurrence | Observation Tips |
---|---|---|
Total Solar Eclipse | August 12, 2026 | Use certified solar viewing glasses; be within the path of totality. |
Partial Lunar Eclipse | March 13-14, 2025 | Safe to view with the naked eye; simply enjoy the gradual shading. |
Annular Solar Eclipse | October 14, 2023 | Always use solar filters; never look directly at the sun. |
Total Lunar Eclipse | Varies; check TimeandDate.com | Capture the event with a camera; no eye protection needed. |
Facts
- Solar eclipses can darken the daytime sky and briefly turn day into night.
- Lunar eclipses last longer, providing ample time for both casual observers and scientists.
- Ancient civilizations saw eclipses as powerful omens or divine messages.
- The Ring of Fire seen during annular solar eclipses is one of nature’s most striking sights.
- Modern technology now allows us to capture these fleeting events in remarkable detail.
Eclipses, whether solar or lunar, offer us a window into the intricate dance of celestial bodies. They are not only dramatic natural phenomena but also serve as critical opportunities for scientific discovery and cultural reflection. Solar eclipses captivate us with their brief moments of darkness and the hidden beauty of the solar corona, while lunar eclipses mesmerize with their gradual transformation and the haunting glow of the Blood Moon. Each eclipse reminds us of the vast, dynamic universe we inhabit and connects us to both our ancient past and our modern quest for knowledge. Embrace these celestial events and join a global community of observers who celebrate one of nature’s most extraordinary shows.
References
-
-
- NASA, “What is the Difference between a Solar Eclipse and a Lunar Eclipse?” accessed Feb. 25, 2025. https://mynasadata.larc.nasa.gov/mini-lessonactivity/what-difference-between-solar-eclipse-and-lunar-eclipse
- NASA, “The Moon & Eclipses,” accessed Feb. 25, 2025. https://science.nasa.gov/moon/eclipses/
- American Astronomical Society, “Shapes of the Sun & Moon In & Out of Eclipse,” accessed Feb. 25, 2025. https://eclipse.aas.org/eclipse-america/sun-moon-shapes
- Vigdis Hocken and Aparna Kher. “What Is a Total Lunar Eclipse? – Sun, Moon & Space.” Time and Date, 20 February 2025, https://www.timeanddate.com/eclipse/total-lunar-eclipse.html Accessed 6 March 2025.
- NASA’s Eclipse Website
-