Terraforming Mars with Tiny Metal Rods: The Future of Making the Red Planet Habitable
- Terraforming Mars involves altering its environment to make it more suitable for Earth-like life.
- A new method proposes using glitter-sized iron and aluminum rods to increase the planet’s temperature by around 30°C.
- Micro-metal rods can be mined from Mars itself, reducing the need to import materials from Earth.
- The concept is 5000 times more efficient than other proposed methods like engineered greenhouse gases.
- Ethical concerns arise around altering another planet’s atmosphere, especially given our limited knowledge of Mars’ deep surface.
Summary
- Terraforming is the process of modifying a planet’s environment to make it more Earth-like.
- Mars currently has an average surface temperature of -65°C, making it inhospitable for Earth-like life.
- Previous proposals for warming Mars included space mirrors and methane pumping, but these were resource-intensive.
- New research by Edwin Kite and colleagues suggests that small iron and aluminum rods could be more efficient.
- These rods are 9 micrometers long and 160 nanometers wide, capable of trapping heat in Mars’ atmosphere.
- Warming effect could raise Mars’ temperature by 30°C, potentially allowing liquid water and supporting microbial life.
- Required materials could be mined directly on Mars, significantly reducing logistical challenges.
- The method would require releasing 700,000 cubic meters of metal per year, equal to 1% of Earth’s annual metal production.
- One challenge is understanding how these rods interact with water in Mars’ atmosphere, which could impact the warming process.
- Ethical considerations include the impact of altering Mars’ atmosphere and whether we should terraform a planet with an unexplored deep surface.
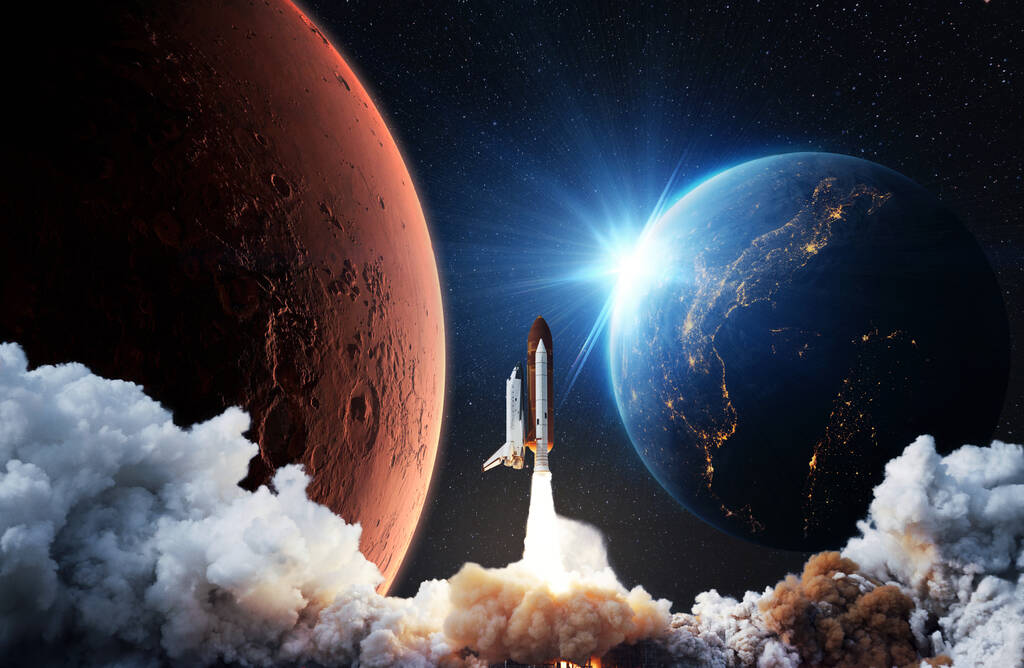
Terraforming Mars with Tiny Metal Rods
Terraforming, the concept of transforming a planet’s environment to resemble Earth’s, has long been a subject of fascination and debate. Mars, our neighboring Red Planet, is the prime candidate for such an undertake. However, the challenges are enormous, given its harsh environment with temperatures averaging -65°C (-85°F). Scientists have proposed various methods to warm Mars, making it more hospitable for life, but most of these methods are resource-intensive and difficult to implement.
A recent study led by Edwin Kite at the University of Chicago presents a novel approach to this problem: using tiny rods of iron and aluminum to warm Mars. This method could be a game-changer in the field of planetary engineering, offering a more efficient and feasible way to terraform Mars.
The Science Behind Terraforming Mars
Mars is a cold, barren planet with a thin atmosphere composed mostly of carbon dioxide. Its surface temperature ranges from -140°C (-220°F) during winter at the poles to 20°C (70°F) during summer at the equator, but the average temperature is a frigid -65°C. The thin atmosphere means that even if the surface heats up during the day, the heat quickly escapes at night.
The idea of terraforming Mars revolves around changing these conditions to create a more Earth-like environment, capable of supporting life. The key challenge is raising the planet’s temperature and atmospheric pressure to allow liquid water to exist, a fundamental requirement for life as we know it.
Previous Proposals
Several ideas have been floated over the years to warm Mars:
- Space Mirrors: Large mirrors in space could reflect sunlight onto Mars’ surface, increasing the temperature. However, the logistics and costs involved in building and deploying such mirrors are staggering.
- Greenhouse Gases: Pumping greenhouse gases like methane into Mars’ atmosphere could trap more heat. But this method would require massive amounts of methane, which would need to be transported from Earth or synthesized on Mars, both of which are currently impractical.
- Nuclear Explosions: Another radical idea involves using nuclear explosions to heat Mars’ poles, releasing trapped CO2 and thickening the atmosphere. This idea is controversial, not least because of the potential dangers and ethical concerns.
Each of these methods has significant drawbacks, making the search for a more efficient solution critical.
The New Approach: Tiny Metal Rods
Edwin Kite and his team propose a new method that could be much more practical and efficient. The idea is to release tiny rods of iron or aluminum, each about 9 micrometers long and 160 nanometers wide, into Mars’ atmosphere. These rods would be mined from Mars’ surface, eliminating the need to transport materials from Earth.
Once released, these rods would be carried by wind into the upper atmosphere, where they would remain for about a decade. Their small size allows them to trap heat effectively, while still allowing sunlight to pass through. The trapped heat would raise the planet’s surface temperature by about 30°C, enough to melt ice and support microbial life.
Kite and his colleagues used climate models to simulate the effects of releasing these rods. Their results showed that the rods could increase the temperature by about 30°C in a matter of months to a decade, depending on how quickly the particles are dispersed. This increase in temperature would also lead to a rise in atmospheric pressure, potentially allowing liquid water to exist on the surface.
The warming effect is critical because it could create conditions suitable for microbial life. Microbes could play a vital role in terraforming Mars, as some bacteria are capable of producing oxygen, further transforming the planet’s atmosphere over time.
Practical Considerations
One of the biggest challenges in terraforming Mars is the sheer amount of material required. However, Kite’s approach is surprisingly efficient. To achieve the necessary warming, only about 700,000 cubic meters of metal rods would need to be released each year. This is equivalent to just 1% of Earth’s total annual metal production, making it a feasible target.
The fact that these materials could be mined directly on Mars is another significant advantage. This reduces the logistical challenges and costs associated with transporting materials from Earth. However, mining on Mars is not without its challenges, and significant technological advancements would be needed to extract and process these metals on the planet.
One of the uncertainties in this method is how the tiny rods would interact with Mars’ atmosphere, particularly with water vapor. There is a possibility that water molecules could cling to the rods, causing them to fall back to the surface as rain. This would reduce the warming effect, as the rods would no longer be in the atmosphere to trap heat.
This interaction needs to be carefully studied, as it could impact the overall effectiveness of the terraforming process. If the rods do indeed fall out of the atmosphere too quickly, alternative strategies might be needed, such as continuously replenishing the rods or finding ways to prevent water from clumping around them.
Ethical Considerations
While the idea of terraforming Mars is exciting, it raises important ethical questions. Mars is a pristine environment, and we know very little about its deep surface and potential for existing life forms. By altering its atmosphere, we could be destroying any chance of discovering native Martian life.
There is also the issue of planetary protection. International agreements currently require that we avoid contaminating other planets with Earth life. Terraforming Mars would almost certainly violate these agreements, as it would involve introducing Earth-based microbes and potentially altering the planet’s environment irreversibly.
Conclusion
Terraforming Mars is one of the most ambitious ideas in human history. It involves changing the planet’s harsh environment to make it more like Earth. The idea of turning a barren, frozen world into a second Earth is exciting. However, it is also very challenging. One new proposal is to use tiny metal rods to warm Mars. This approach seems promising and could help make the dream of terraforming Mars come true.
However, before we can proceed, we must carefully consider the ethical implications and ensure that we are not causing irreversible harm to a planet we are only just beginning to understand. With careful planning, international collaboration, and ongoing research, terraforming Mars could one day become a reality, offering a new frontier for human exploration and habitation.
Sources:
- Science Advances DOI: 10.1126/sciadv.adn4650: https://dx.doi.org/10.1126/sciadv.adn4650
- Manoj Joshi, University of East Anglia: https://research-portal.uea.ac.uk/en/persons/manoj-joshi
- Edwin Kite, University of Chicago: https://geosci.uchicago.edu/people/edwin-kite/
- Space mirrors for terraforming: https://www.newscientist.com/article/dn10573-space-mirrors-could-create-earth-like-haven-on-mars/
- Terraforming Mars and carbon dioxide: https://www.newscientist.com/article/2175414-terraforming-mars-might-be-impossible-due-to-a-lack-of-carbon-dioxide/
Hashtags
#TerraformingMars, #MarsExploration, #PlanetaryScience, #SpaceExploration, #MarsTerraforming, #SpaceScience, #FutureOfSpace, #Astrobiology