Neutron Star Collisions and the Early Universe: A Remarkable Cosmic Parallel
The phenomenon of neutron star collisions, resulting in powerful explosions known as kilonovae, holds crucial clues about the early universe. These collisions produce a plasma state reminiscent of the early Big Bang era, create heavy elements through nucleosynthesis, and have led to groundbreaking insights into the nature of atomic formation. The kilonova event AT2017gfo provided an unprecedented glimpse into the universe’s material evolution and the formation of a black hole, shedding light on cosmic processes that took place billions of years ago.
Summary
- Neutron stars are highly dense stellar remnants, packing massive amounts of matter into small volumes.
- When two neutron stars collide, the resulting kilonova explosion releases vast energy, creating conditions similar to those of the early universe.
- The kilonova AT2017gfo, observed in 2017, was the first confirmed observation of its kind, providing critical data on heavy element formation.
- This explosion created elements through the rapid neutron capture process (r-process), leading to the formation of gold, platinum, and uranium.
- By analyzing spectra from telescopes around the globe and Hubble in orbit, researchers watched as atoms formed in real-time, for the first time.
- The event also suggests the creation of a black hole, showcasing the formation of extreme celestial objects in neutron star mergers.
- Researchers believe kilonovae contribute significantly to the universe’s heavy elements, pushing forward our understanding of nucleosynthesis.
Main Article
Neutron stars represent some of the densest objects in the universe, remnants of massive stars that have undergone supernova explosions. They’re typically about 20 kilometers in diameter but pack the mass of several suns, resulting in extreme gravitational fields. When two neutron stars collide, they produce a phenomenon known as a kilonova — an explosion that is among the most energetic events in the cosmos. This event releases elements and radiation that help us better understand the universe’s origins and development, much like the Big Bang itself.
A Glimpse of the Early Universe
The process following a neutron star collision and the subsequent kilonova explosion shares remarkable parallels with conditions just after the Big Bang. At that time, the universe was a hot, dense plasma where atomic nuclei and electrons were separated. In a similar fashion, neutron star collisions release enough energy to create a plasma of detached electrons and atomic nuclei. However, as the plasma cools, these particles can combine to form atoms through a process called nucleosynthesis.
“For the first time, we see the creation of atoms in a cosmic event,” remarked Rasmus Damgaard, Ph.D. student at the Cosmic DAWN Center. This discovery demonstrates the process of atomic formation and material cooling that characterizes both kilonovae and the early universe.
Understanding Nucleosynthesis
Nucleosynthesis — the formation of atomic nuclei from protons and neutrons — occurs in various astrophysical environments. There are three main processes:
- Slow neutron capture (s-process)
- Proton process (p-process)
- Rapid neutron capture (r-process)
In kilonovae, rapid neutron capture (r-process) is dominant, which is responsible for producing many of the universe’s heaviest elements, including gold, platinum, and uranium.
Below is a table showing these three nucleosynthesis processes and their primary characteristics.
Process | Environment | Key Elements Produced |
---|---|---|
s-process | Stellar environments | Copper, silver, lead |
p-process | Supernova environments | Selenium, molybdenum, tellurium |
r-process | Kilonova environments | Gold, platinum, uranium |
The Historic Observation of AT2017gfo
The kilonova event AT2017gfo marked a breakthrough in astrophysics, as it allowed scientists to witness nucleosynthesis in real time. Discovered in 2017, this kilonova was observed in conjunction with gravitational waves from the event GW170817, detected by LIGO. It was a defining moment because the gravitational wave detection provided additional information about the physical conditions during the collision, leading to the most detailed analysis of a kilonova to date.
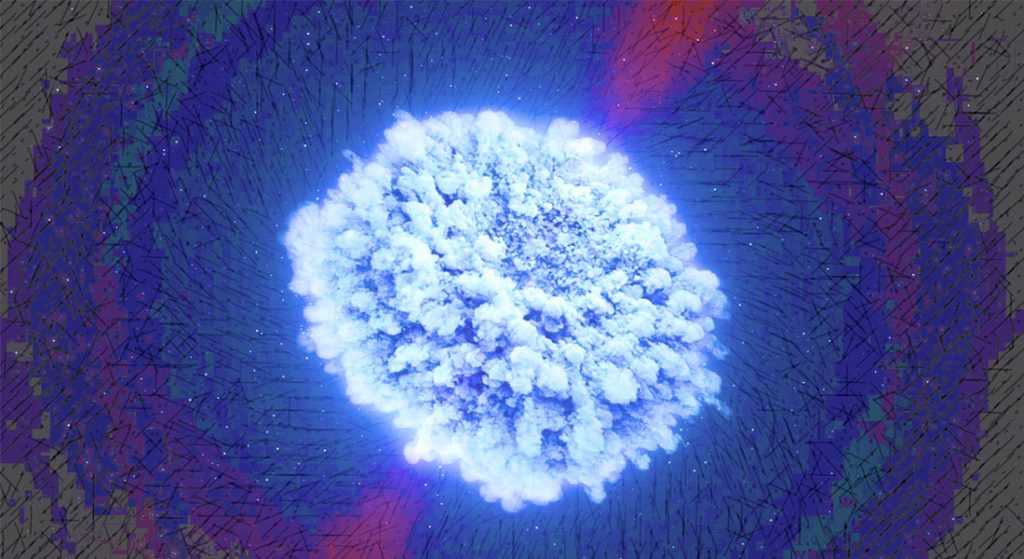
A neutron star is an extremely dense star that forms after a supernova explosion. A supernova is a powerful explosion that happens when a star dies. The Big Bang is a scientific theory explaining how the Universe began. It started with a small, hot, and dense point that expanded rapidly.
Challenges in Observation
Kilonovae, despite their energy output, are transient and fade within days, making them challenging to observe. The Earth’s rotation limits telescope views to certain times, so researchers had to piece together data from multiple sources worldwide, including telescopes in Australia, South Africa, and the Hubble Space Telescope in low-Earth orbit. “The viewing angle of individual telescopes is blocked by Earth’s rotation,” noted Albert Sneppen from the Cosmic Dawn Center. Combining observations from different sites provided a fuller view of the kilonova’s evolution.
Revealing Atomic Synthesis through Spectroscopy
By analyzing the spectra collected from AT2017gfo between 0.5 and 9.4 days after the event, researchers focused on optical and near-infrared (NIR) wavelengths, as shorter wavelengths like X-rays and ultraviolet (UV) were opaque at that stage. These spectra revealed the formation of elements like strontium, tellurium, lanthanum, cesium, and yttrium. These findings were derived by studying a P Cygni spectral line — an indicator of an expanding shell of gas around the kilonova — which provided data on velocity, density, and other parameters of the ejecta.
Observation Wavelength | Importance | Notable Elements Observed |
---|---|---|
Optical | High visibility in early cooling stages | Strontium |
Near-infrared (NIR) | Penetrates thick ejecta to reveal more details | Lanthanum, Tellurium |
Cosmic Implications: Heavy Elements and Black Holes
Neutron star collisions do more than create heavy elements; they also often result in black hole formation. Following the AT2017gfo explosion, researchers identified evidence suggesting the creation of one of the smallest black holes observed. The event’s gravitational wave signature, GW170817, was detected by LIGO and provided data that supported the formation of a black hole, though there is still speculation about the possibility of a magnetar — a type of neutron star with an ultra-strong magnetic field — being involved.
“The matter expands so fast and gains in size so rapidly that it takes hours for the light to travel across the explosion. Observing the farthest end of the fireball takes us further back in the history of the explosion,” said Kasper Heintz, assistant professor at the Niels Bohr Institute.
Kilonovae as Cosmic Laboratories
Kilonovae serve as natural laboratories where extreme physics plays out on a cosmic scale. Their environments allow scientists to study nuclear reactions that are impossible to replicate on Earth. The heavy elements produced, especially gold and platinum, highlight the importance of kilonovae in enriching the galaxy with these rare elements.
Facts About Neutron Star Collisions and Kilonovae
- Small but Mighty: A neutron star is about the size of a city, yet it can weigh as much as 2.5 times the sun.
- Blinding Brightness: Kilonovae can outshine entire galaxies for a brief period.
- Gold in Space: Neutron star collisions are responsible for creating around 10 Earth masses of gold in a single explosion.
The Role of Advanced Telescopes in Kilonova Research
The study of neutron star collisions has advanced significantly due to telescopes like Hubble and LIGO. The ability to detect gravitational waves has enabled astronomers to pinpoint collision events with accuracy. The multi-telescope approach, as seen in the study of AT2017gfo, allowed scientists to observe these high-energy events from multiple angles.
The study of neutron star collisions and kilonovae provides profound insights into the early universe and the formation of elements essential to life on Earth. The event AT2017gfo stands as a testament to the strides made in astrophysics, unveiling the mysteries of atomic synthesis and black hole formation. As technology advances, we are likely to witness even more detailed observations of these celestial events, furthering our understanding of the cosmos.