The Quasar That Brought Light to the Universe’s Dark Ages
The quasar J1429+5447, located 12 billion light-years away, provides valuable insights into the universe’s early evolution. By studying its powerful X-ray emissions and rapid variability, astronomers believe it played a significant role in ending the Dark Ages and initiating the Era of Reionization.
Summary
- The universe began 13.8 billion years ago with the Big Bang, transitioning from the Dark Ages to the epoch of reionization.
- The Dark Ages were a period when the universe lacked visible light, and neutral hydrogen dominated the cosmos.
- J1429+5447, a quasar located 12 billion light-years away, was discovered to play a critical role in the reionization process.
- Quasars are powered by supermassive black holes that release extreme amounts of energy, including X-rays and ultraviolet radiation.
- NuSTAR and Chandra X-ray telescopes studied J1429+5447, revealing intense and rapid X-ray variability over a short 4-month period.
- Professor Meg Urry from Yale University explained how the quasar’s jets pointed directly toward Earth, amplifying their observed brightness due to Einstein’s theory of special relativity.
- The intense radiation from quasars like J1429+5447 may have reionized hydrogen, ending the universe’s Dark Ages and making it transparent.
- The discovery underlines the importance of quasars in shaping the early universe’s structure.
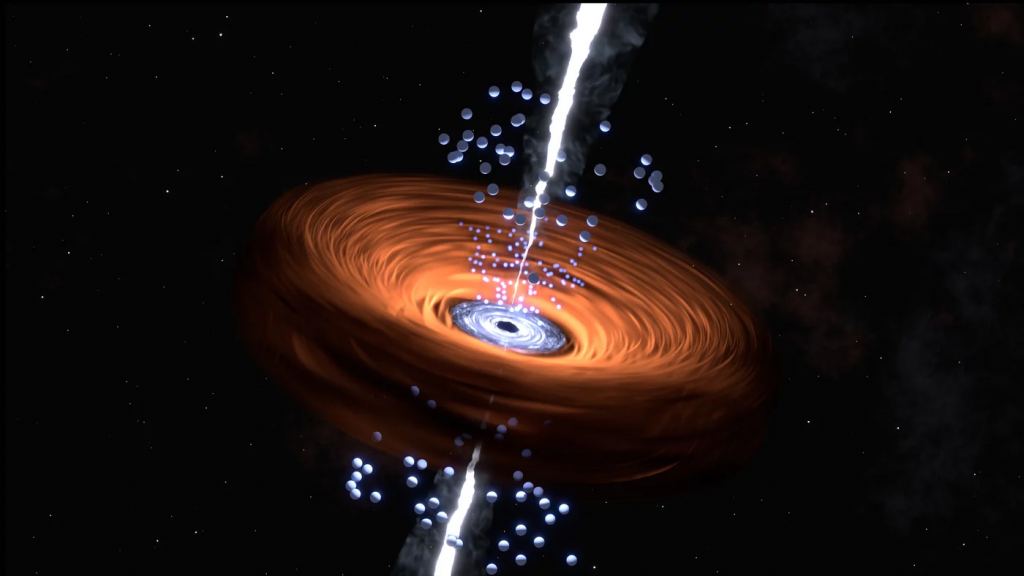
Introduction
After the Big Bang, the universe entered a mysterious period known as the Dark Ages. For hundreds of millions of years, no light existed to illuminate the vast expanse of space. This changed with the advent of the epoch of reionization, where the universe’s first stars and galaxies began forming. A key question has puzzled scientists for decades: what caused the reionization?
In a groundbreaking discovery, a team of researchers from Yale University identified a distant quasar, J1429+5447, as one of the celestial objects that played a vital role in ending the Dark Ages. By pumping out vast amounts of X-ray radiation, quasars like this one may have catalyzed the transition into a luminous universe.
The Early Universe: From Darkness to Light
The universe began with the Big Bang approximately 13.8 billion years ago, starting as an incredibly hot and dense singularity. Over time, it expanded and cooled, allowing the formation of light elements like hydrogen and helium. During the first few hundred thousand years, light was trapped in a dense fog of neutral hydrogen.
Around 380,000 years after the Big Bang, the Cosmic Microwave Background (CMB) emerged, marking the end of the Dark Ages. As the universe continued to expand, gravity pulled matter together to form the first stars and galaxies. These early structures emitted high-energy radiation that ionized hydrogen, making the universe transparent and giving rise to the Epoch of Reionization.
This period of transformation laid the foundation for the development of large-scale structures such as galaxies, clusters, and eventually our solar system, which formed approximately 4.6 billion years ago.
Observing the Quasar J1429+5447
The quasar J1429+5447, located in the constellation of Lyra, offers a window into the universe’s early days. Its light has taken 12 billion years to reach Earth, meaning astronomers observe it as it was just 1.6 billion years after the Big Bang.
Quasars, or quasi-stellar objects, are powered by supermassive black holes at the centers of galaxies. As matter falls into these black holes, it forms an accretion disk that releases immense amounts of radiation across the electromagnetic spectrum, including visible light, X-rays, and ultraviolet (UV) radiation.
Using the NuSTAR X-ray telescope, the team of researchers observed the quasar’s behavior over four months. They compared their findings with earlier studies conducted using the Chandra X-ray Observatory. Remarkably, the quasar’s X-ray emissions doubled in intensity during this short period.
The Role of Quasars in Reionization
Quasars like J1429+5447 are believed to have been instrumental in reionizing the universe. Their intense radiation ionized neutral hydrogen, ending the Dark Ages and enabling light to travel freely through space.
According to Professor Meg Urry, a leading astrophysicist and co-author of the study, the quasar’s jets likely pointed directly toward Earth. This alignment caused the observed brightness to increase dramatically due to the effects of Einstein’s theory of special relativity.
“The level of X-ray variability in terms of intensity and rapidity is extreme. It is almost certainly explained by a jet pointing toward us – a cone in which particles are transported up to a million light-years away from the central, supermassive black hole.” – Professor Meg Urry
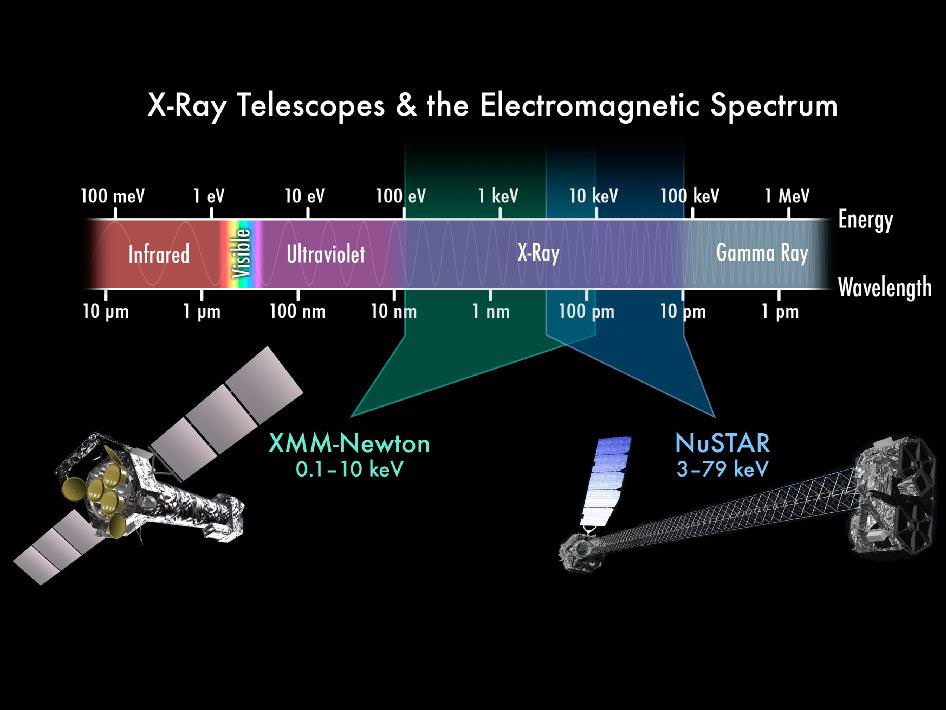
Observational Tools and Techniques
The discovery of J1429+5447 relied on advanced space telescopes capable of detecting high-energy X-rays. Two key instruments were used:
Telescope | Key Features and Observations |
---|---|
NuSTAR | Detects high-energy X-rays with exceptional sensitivity and clarity. Used to observe the quasar’s rapid variability over a 4-month period. |
Chandra X-ray Observatory | Provides high-resolution X-ray imaging and spectroscopy. Earlier observations of the quasar served as a reference for comparison. |
The spectral ranges of these telescopes, shown in the table below, highlight their ability to capture crucial data from distant quasars.
Telescope | Spectral Range (keV) | Primary Purpose |
---|---|---|
NuSTAR | 3 – 79 keV | High-energy X-ray astronomy |
Chandra | 0.1 – 10 keV | X-ray imaging and spectroscopy |
These tools have enabled astronomers to study the role of quasars in reionizing the universe with unprecedented detail.
Implications of the Discovery
The discovery of J1429+5447 has profound implications for our understanding of the early universe. It provides evidence that quasars were among the most influential objects in ending the Dark Ages.
By emitting high-energy radiation, quasars ionized vast amounts of neutral hydrogen, allowing light to permeate the cosmos. This process also contributed to the formation of galaxies, stars, and other large-scale structures.
Additionally, the study highlights the importance of jet alignment in amplifying the observed brightness of quasars. The findings support the idea that jets play a critical role in transporting energy across vast distances, influencing the evolution of the surrounding environment.
Facts About Quasars
- Quasars are among the most luminous objects in the universe, capable of outshining entire galaxies.
- The energy output of a single quasar can equal that of a trillion suns.
- The first quasar was discovered in 1963 by astronomer Maarten Schmidt.
- Quasars are powered by supermassive black holes with masses ranging from millions to billions of times that of the Sun.
- The jets emitted by quasars can extend over millions of light-years, influencing nearby galaxies.