A Triple Star System: Hubble’s New Discovery
Key Takeaway
Triple star systems, where three stars orbit each other, give us special insights into how stars move and form. These systems are interesting because of their complex orbits and what they can teach us about the universe as a whole.
Summary
- Triple star systems consist of three stars bound by gravity.
- Formation theories include fragmentation of a molecular cloud or gravitational capture.
- Orbital Movement are complex and can involve hierarchical arrangements.
- Types of triple systems vary based on the stars’ mass and orbit configuration.
- Observations are made using advanced telescopes and astrometric techniques.
- Stability of these systems is a subject of ongoing research.
- Notable triple star systems include Alpha Centauri and Polaris.
- New discoveries such as the HP Tau system show the continued relevance of Hubble Space Telescope.
- Implications for exoplanetary systems and astrobiology are significant.
- Future research will leverage next-gen telescopes for deeper insights.

The Mysteries of Triple Star Systems
Triple star systems, where three stars are held together by gravity and orbit each other, are some of the most fascinating things in space science. These star groupings make us rethink what we know about how stars form, move, and change over time. In this article, we will look into the details of triple star systems, including how they form, the different types, how they move, and the tools scientists use to study them. We will also talk about new findings, like Hubble’s recent discovery of a new triple star system, HP Tau.
Notable Triple Star Systems
Some of the most famous triple star systems have provided valuable insights into stellar dynamics and evolution.
- Alpha Centauri: This nearby system consists of Alpha Centauri A and B, which form a close binary, and Proxima Centauri, a red dwarf that orbits the pair at a much greater distance. Proxima Centauri is the closest known star to the Sun.
- Polaris: Known as the North Star, Polaris is a triple star system with a close binary pair and a more distant companion. The primary star, Polaris A, is a supergiant, making this system a key reference point in celestial navigation.
- HP Tau: The Hubble Space Telescope recently captured a stunning image of this new triple star system. Located 550 light-years away in the Taurus constellation, HP Tau consists of HP Tau, HP Tau G2, and HP Tau G3. These stars are incredibly young, with HP Tau being a T Tau star, still surrounded by its protoplanetary disk.
Hubble’s Contribution: The Discovery of HP Tau
In a world shifting its focus from the Hubble Space Telescope to the James Webb Space Telescope, Hubble continues to prove its worth. Recently, it captured an amazing image of the triple star system HP Tau, HP Tau G2, and HP Tau G3. These stars, located in a reflection nebula in Taurus, are extremely young. HP Tau is so young it hasn’t started fusing hydrogen yet and is only about 10 million years old.
Hubble, launched in 1990, orbits Earth at an altitude of around 547 kilometers. It collects light with its 2.4m mirror and directs it to instruments that record and analyze it. This recent image from Hubble shows a reflection nebula 550 light-years away, made of interstellar dust reflecting light from nearby stars, giving it a characteristic blue hue.
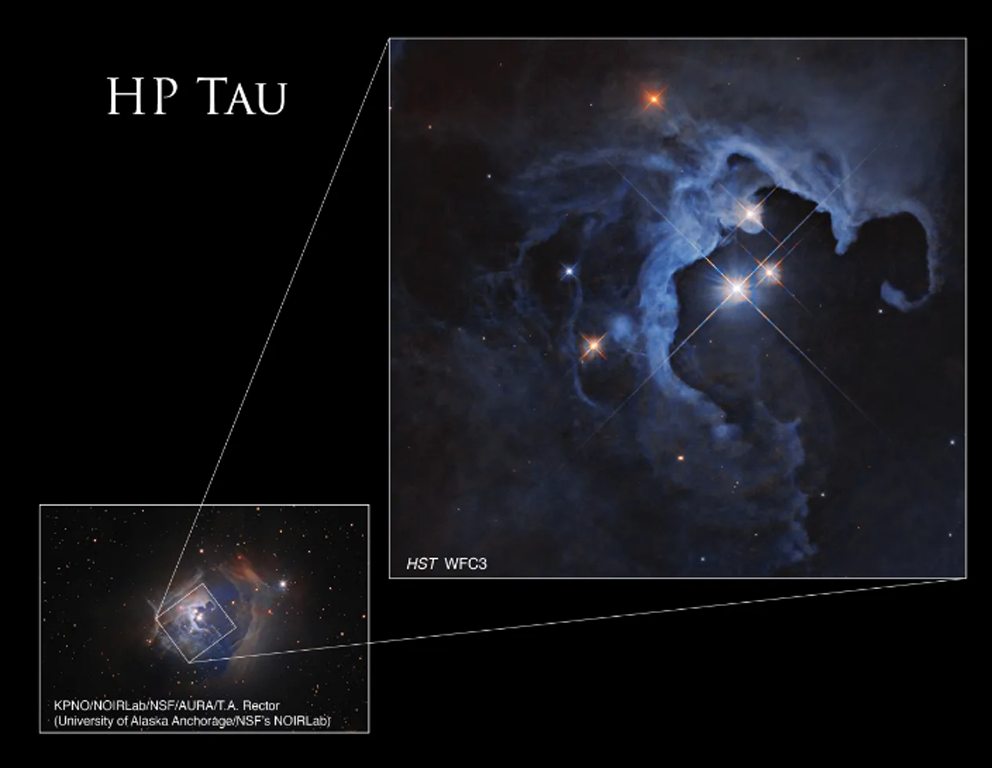
NASA, ESA, G. Duchene (Universite de Grenoble I); Image Processing: Gladys Kober (NASA/Catholic University of America); Inset: KPNO/NOIRLab/NSF/AURA/T.A. Rector (University of Alaska Anchorage/NSF’s NOIRLab)
Formation of Triple Star Systems
Triple star systems can form through several mechanisms, each offering a unique glimpse into the processes that shape our universe.
- Fragmentation of a Molecular Cloud: One primary theory suggests that a single large molecular cloud can fragment into multiple cores during its collapse, each core forming a star. If the fragmentation process is particularly active, it can lead to the creation of a multiple star system.
- Gravitational Capture: Another possible formation mechanism is gravitational capture. In regions of space with high stellar density, a close encounter between stars can result in one star being captured by an existing binary system, forming a triple system.
- Disk Fragmentation: A circumstellar disk around a newly formed star can become gravitationally unstable, fragmenting to form additional stars. This process can also lead to the formation of multiple star systems.
Orbital Movement
The orbital movement of triple star systems are complicated and often involve hierarchical arrangements, where one pair of stars orbits each other closely while the third star orbits at a greater distance. This hierarchical structure helps maintain stability within the system.
Types of Orbits
- Hierarchical Triple Systems: The most common arrangement, where two stars form a close binary system, and the third star orbits this pair at a much greater distance.
- Non-Hierarchical Triple Systems: In these rare configurations, all three stars have similar distances and dynamically interact with each other in a more chaotic manner.
Types of Triple Star Systems
Triple star systems can be classified based on the mass and orbital configuration of the stars involved. Here are a few common types:
- Spectroscopic Triples: These systems are identified through their spectral lines. The stars are so close that their individual spectra overlap, and their presence is inferred through shifts in these lines due to their orbital motion.
- Visual Triples: These systems can be resolved through telescopes, allowing direct observation of their individual components and their motions.
- Eclipsing Triples: In these systems, the stars pass in front of each other from our perspective, causing periodic dips in brightness that reveal details about their orbits and sizes.
Observational Techniques
Studying triple star systems requires advanced observational techniques and instruments. Astronomers use a combination of methods to gather data on these complex systems.
- Astrometry: Precise measurements of the stars’ positions and movements over time help determine their orbits and masses.
- Spectroscopy: Analyzing the light spectra from these stars reveals their composition, temperatures, and radial velocities, which can be used to infer orbital parameters.
- Interferometry: This technique combines light from multiple telescopes to achieve higher resolution, allowing astronomers to resolve close binary systems and their tertiary companions.
Stability and Evolution
The stability of triple star systems is a subject of ongoing research. Factors such as the masses of the stars, their orbital distances, and their interactions determine whether the system remains stable over long periods or eventually breaks apart.
Stability Criteria
- Hierarchical Structure: Systems with a hierarchical structure are more likely to remain stable because the gravitational interactions between the stars are less chaotic.
- Resonances: Orbital resonances, where the stars’ orbits are in integer ratios, can enhance stability by reducing chaotic interactions.
- Mass Ratios: Systems where one star is significantly more massive than the others tend to be more stable, as the massive star can dominate the gravitational dynamics.
Implications for Exoplanetary Systems
The study of triple star systems has significant implications for the search for exoplanets and the understanding of planetary formation.
- Habitable Zones: The complex gravitational interactions in triple star systems can affect the habitable zones where life might exist. Planets in these systems might experience varying levels of radiation and gravitational forces, impacting their potential habitability.
- Planetary Formation: Understanding how planets form and evolve in multi-star systems helps refine models of planetary system formation. Triple star systems challenge existing theories and push the boundaries of our knowledge.
- Protoplanetary Disks: Hubble’s observation of HP Tau was part of an investigation into protoplanetary disks. These disks are believed to be the progenitors to planetary systems, providing insight into the early stages of planet formation.
Future Research and Exploration
Advancements in technology will continue to drive the study of triple star systems forward. Next-generation telescopes and space missions promise deeper insights and more detailed observations.
- James Webb Space Telescope (JWST): With its advanced infrared capabilities, the JWST will allow astronomers to peer through dust clouds and study the formation and evolution of triple star systems in unprecedented detail.
- Ground-Based Observatories: Facilities like the Extremely Large Telescope (ELT) will provide higher resolution images and spectra, aiding in the study of these complex systems.
- Space Missions: Proposed missions like the Laser Interferometer Space Antenna (LISA) will detect gravitational waves from triple star systems, offering a new way to study their dynamics.
Table 1: Notable Triple Star Systems
System | Components | Distance from Earth (light-years) | Characteristics |
---|---|---|---|
Alpha Centauri | Alpha Centauri A, B, Proxima | 4.37 | Closest triple system to Earth, includes Proxima Centauri |
Polaris | Polaris A, B, and C | 433 | North Star, includes a supergiant and two smaller stars |
Algol | Algol A, B, and C | 93 | Eclipsing binary with a third star, known as the “Demon Star” |
Castor | Castor A, B, and C | 51 | Part of a sextuple star system, with three close binaries |
HP Tau | HP Tau, HP Tau G2, and HP Tau G3 | 550 | Young stars in a reflection nebula, observed by Hubble |
Table 2: Methods of Observing Triple Star Systems
Method | Description | Advantages | Limitations |
---|---|---|---|
Astrometry | Measures positions and motions of stars | High precision in determining orbits | Requires long-term observation |
Spectroscopy | Analyzes light spectra to determine composition and motion | Reveals detailed information about stars’ properties | Limited by spectral resolution and signal |
Interferometry | Combines light from multiple telescopes for higher resolution | Resolves close binaries and distant companions | Complex setup and calibration required |
Photometry | Measures brightness variations | Detects eclipsing binaries and transits | Sensitivity to external light interference |
Triple star systems are a fascinating area of study in astrophysics, They help us learn a lot about how stars move and form. These systems have tricky patterns in how they move around each other, and they teach us a lot about planets outside our solar system. They make us rethink what we know and help us learn more about space. As our tools get better, we’ll learn even more about these mysterious groups of stars. Recently, Hubble found a new triple star system called HP Tau. This shows that even older telescopes are still important for discovering new things about space.
Hashtags
#Astrophysics, #TripleStarSystems, #Astronomy, #SpaceExploration, #StellarDynamics, #Exoplanets, #JamesWebbSpaceTelescope, #AlphaCentauri, #Polaris, #SpaceResearch, #HubbleSpaceTelescope, #HPTau #A Triple Star System
Reference
- NASA. (2024). Hubble Views the Dawn of a Sun-like Star. Retrieved from NASA