Can Hot Jupiters Co-Exist with Other Planets? New Research Explains
Hot Jupiters, long believed to be solitary exoplanets due to their violent migratory paths, have now been discovered coexisting with other planets in the same system. This groundbreaking finding challenges traditional theories of planetary formation and migration, paving the way for an alternative understanding of how these massive gas giants interact with other celestial bodies.
Summary
- Hot Jupiters are gas giants that orbit their host stars at extreme proximity, completing an orbit in just days or hours.
- Due to their close orbits, they experience intense radiation, causing their atmospheres to reach scorching temperatures and expand significantly.
- Traditional models of planetary migration suggested that Hot Jupiters formed farther out and migrated inward, scattering or destroying any neighboring planets in the process.
- New research from a team of astronomers at the University of Geneva (UNIGE), in collaboration with UNIBE and UZH, has discovered a system where a Hot Jupiter coexists with a Super-Earth and another gas giant.
- Observations from WASP-132, a star located over 400 light-years away, reveal a Hot Jupiter with an orbital period of 7.1 days and a mass of 0.41 Jupiter masses.
- The HARPS spectrograph at the La Silla Observatory identified a Super-Earth in the same system, with a mass six times that of Earth.
- The Gaia satellite is refining measurements of the star system to confirm the planetary masses and orbits more precisely.
- This discovery suggests that Hot Jupiters can have “cooler” and less violent migratory paths, preserving their planetary neighbors.
- Further exploration and study of similar systems will help refine current migration models and deepen our understanding of exoplanetary dynamics.
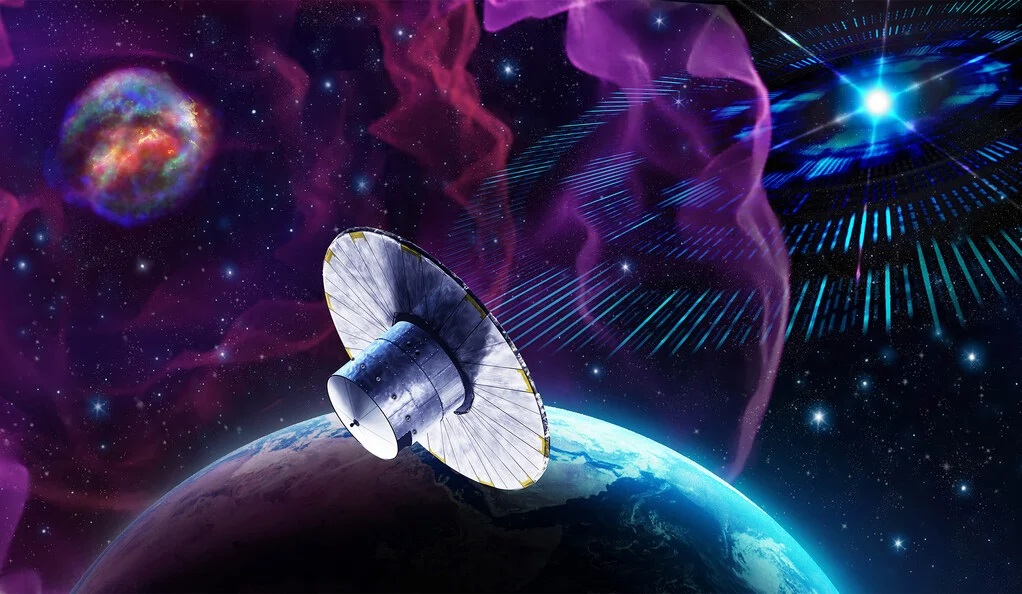
Introduction
Hot Jupiters are one of the most fascinating and puzzling types of exoplanets discovered in recent years. These gas giants, similar in size and composition to our own Jupiter, defy traditional planetary formation models by orbiting perilously close to their stars. Their proximity subjects them to extreme temperatures, swelling their atmospheres and making them a unique class of celestial objects.
Traditionally, Hot Jupiters were thought to have formed in the cooler outer regions of their solar systems and later migrated inward, causing chaos along the way. They were believed to eject or destroy any neighboring planets in their path. However, a recent study challenges this notion, presenting the first evidence of a Hot Jupiter coexisting with other planets in a stable system.
This revelation not only expands our understanding of exoplanetary systems but also raises intriguing questions about the migration and formation of these enigmatic planets.
What Are Hot Jupiters?
Hot Jupiters are gas giants that resemble Jupiter in mass and composition but differ dramatically in their orbital characteristics. Unlike Jupiter, which takes 12 years to complete an orbit, Hot Jupiters orbit their stars in just days or even hours.
These planets are subjected to intense stellar radiation, causing their atmospheres to reach extreme temperatures exceeding 1,000°C. This heat also leads to atmospheric expansion, making some Hot Jupiters appear significantly larger than expected.
The table below summarizes key characteristics of Hot Jupiters:
Characteristic | Details |
---|---|
Orbital Period | Days to hours |
Temperature | Over 1,000°C |
Atmospheric Composition | Hydrogen and helium |
Migration Hypothesis | Formed far from the star, migrated inward |
The Migration Conundrum
According to established theories of planetary formation, inner planets are composed of denser materials, while outer planets are primarily made of lighter elements. This is because lighter elements are pushed outward by the energy from the forming star.
The presence of Hot Jupiters so close to their stars contradicts this model, suggesting they formed in the cooler outer regions and later migrated inward. However, this migration process was believed to be catastrophic, leaving the Hot Jupiter as the sole survivor in its system.
An artist’s impression of a Hot Jupiter forming and migrating inward can be seen here.
A Paradigm Shift: WASP-132 System
Recent observations by a team of astronomers at UNIGE and its partners have upended the traditional understanding of Hot Jupiters. They discovered a multiple planetary system orbiting the star WASP-132, located over 400 light-years away.
The system includes:
- A Hot Jupiter with a mass of 0.41 Jupiter masses and an orbital period of 7.1 days.
- A Super-Earth with a mass six times that of Earth, located in an inner orbit.
- Another gas giant in an outer orbit, resembling conventional gas giants like Jupiter.
This discovery was made using photometric measurements and the HARPS spectrograph at the La Silla Observatory in Chile. Further refinements are being conducted using the Gaia satellite, which measures the star’s minute positional changes caused by its planets.
An artist’s impression of the Gaia spacecraft can be viewed here.
Implications of the Discovery
This finding has profound implications for our understanding of planetary migration and system stability. It suggests that Hot Jupiters may not always have destructive migration paths. Instead, they could follow a more “gentle” trajectory that allows other planets to coexist.
As the researchers refine their measurements and analyze similar systems, we may uncover new insights into the dynamics of planetary systems and the factors that influence their formation and evolution.
Facts About Hot Jupiters
- Hot Jupiters are often referred to as “roasters” due to their extreme temperatures.
- Some Hot Jupiters experience “atmospheric escape,” where their atmospheres are stripped away by stellar radiation.
- They are easier to detect using the transit method because their large size blocks more light when passing in front of their star.
Future Research Directions
The discovery of the WASP-132 system opens the door to several exciting research avenues:
- Refining Migration Models: Current theories need to account for less violent migration paths.
- Exploring Similar Systems: Identifying other Hot Jupiter systems with multiple planets will help validate the findings.
- Long-Term Observations: Continuous monitoring of the WASP-132 system and others like it will provide deeper insights into their dynamics.
The table below highlights the key tools used in these investigations:
Instrument | Purpose |
---|---|
HARPS Spectrograph | Measures radial velocity of stars |
Gaia Satellite | Tracks positional changes of stars |
Photometric Measurements | Detects planetary transits |