How Nuclear Propulsion Could Enable Crewed Missions to Titan
A crewed mission to Titan—Saturn’s largest moon—may become possible using advanced nuclear propulsion systems. By leveraging concepts like nuclear-thermal propulsion, nuclear-electric propulsion, and emerging fusion drives, transit times could be cut to under a year one-way, reducing health risks and enabling humanity’s next giant leap into the outer Solar System.
Summary
- Titan was first closely observed by Pioneer 11 in 1979, revealing its hazy orange haze
- Voyager and Cassini–Huygens missions mapped Titan’s nitrogen-rich atmosphere and organic surface
- Titan’s methane cycle mirrors Earth’s water cycle, hinting at prebiotic chemistry
- NASA’s Dragonfly rotorcraft, launching in 2028 and arriving in 2034, will hunt for biosignatures
- Explore Titan, a non‑profit, proposes crewed missions using nuclear-fission propulsion
- LPSC 2025 paper by O’Hara & Fernandez‑Tous outlines reactor sizing for Titan voyages
- Nuclear‑Thermal Propulsion (NTP) could match Mars mission designs but needs scaling for Titan
- Copernicus NTP concept may cut one-way travel to ~150 days but raises radiation concerns
- Nuclear‑Electric Propulsion (NEP) like VASIMR offers high efficiency and transit times under 150 days
- Direct Fusion Drives (DFD) promise multi‑year round trips with heavy payloads, pending reactor development
- Crew health hinges on limiting microgravity and cosmic radiation exposure
- Nuclear propulsion could unlock human exploration of distant worlds beyond Mars
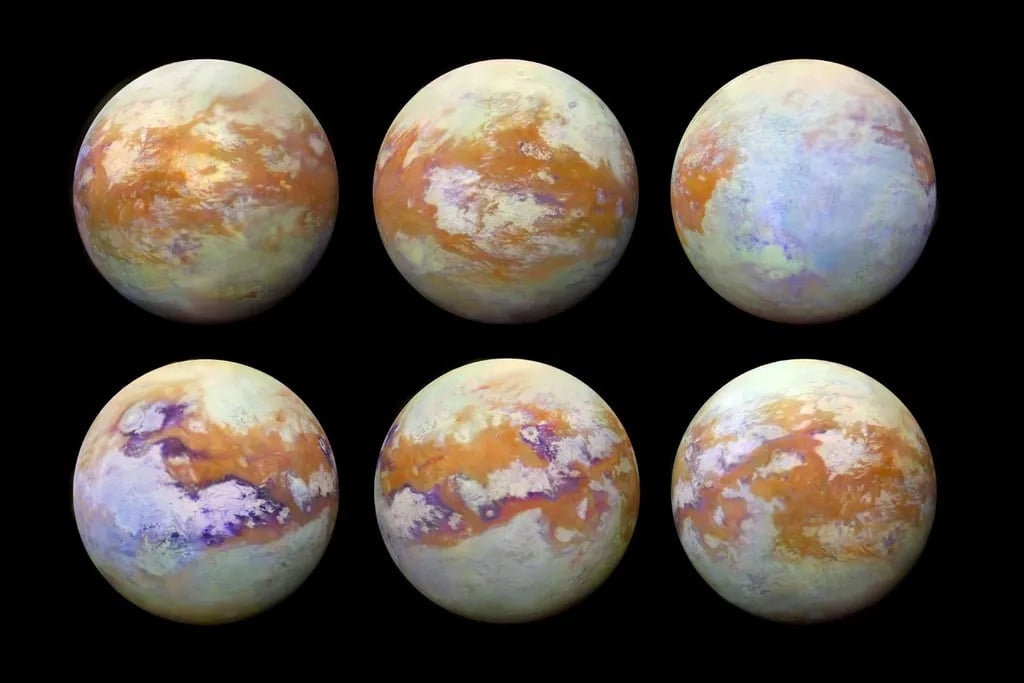
Introduction
Saturn’s moon Titan stands out in the Solar System for its dense, orange‐tinted skies and organic chemistry. First visited by Pioneer 11 in November 1979, Titan’s mysterious haze prompted follow‑up reconnaissance by the Voyager probes and the landmark Cassini–Huygens mission, which revealed a thick, nitrogen‑rich atmosphere and liquid methane–ethane lakes on its surface. Titan is the only body besides Earth with a substantial atmosphere, composed of about 98 % nitrogen and 2 % methane, creating a cycle of evaporation and rain akin to Earth’s water cycle. These discoveries fuel the quest to find life beyond our planet.
Exploring Titan with robots begins with Dragonfly, a nuclear‑powered rotorcraft that will launch in July 2028 and arrive in 2034 to probe for prebiotic chemistry at multiple sites. Yet many scientists ask: Could humans ever set foot on Titan? A recent study by Explore Titan, Inc. explores how nuclear-fission propulsion might carry a crew there in under two years one‑way.
Advances in Nuclear Propulsion
Research into nuclear propulsion splits into two main camps: nuclear-thermal propulsion (NTP) and nuclear-electric propulsion (NEP). NTP systems, like those outlined in NASA’s Design Reference Architecture 5.0 (DRA 5.0), use a uranium‑235 reactor to heat hydrogen propellant to high exhaust velocities. A crewed Mars mission based on DRA 5.0 envisions a 56‑metric‑ton spacecraft capable of a 375‑day round trip . Scaling this for Titan requires more propellant and higher thrust.
Copernicus, a larger NTP concept from NASA Glenn, ups propellant capacity to 172 metric tons, potentially bringing one‑way transit down to 150–220 days. However, longer exposure to cosmic rays on a multi‑month voyage poses serious health risks. Increasing propellant further could shorten the trip to 90 days, but the added mass drives up cost and complexity.
NEP systems generate electricity via a reactor and power electric thrusters. Concepts like VASIMR (Variable Specific Impulse Magnetoplasma Rocket) have shown potential to cut Titan transit times to under 150 days by using magnetic fields to accelerate plasma jets. NEP’s higher efficiency can reduce propellant needs, but the power‑to‑thrust ratio remains a challenge for heavy crewed ships.
Table 1: Propulsion Options Overview
Propulsion Type | Key Feature | One‑Way Transit |
---|---|---|
NTP (DRA 5.0) | High thrust, heavy hydrogen fuel | ~375 days to Mars |
Copernicus NTP | Extended propellant capacity | 150–220 days to Titan |
NEP (VASIMR) | High efficiency electric thrust | ~149 days to Titan |
Emerging Fusion Solutions
Beyond fission, fusion‑driven rockets may revolutionize deep‑space travel. Studies at Princeton Satellite Systems demonstrate how a Direct Fusion Drive (DFD) could ferry a 1,000 kg payload to Titan in under 2.6 years—twice as fast as Dragonfly’s seven‑year flight. Fusion engines promise both thrust and electrical power from the same reactor, potentially powering life‑support and onboard systems.
Crew Health and Mission Design
Long voyages in microgravity can degrade muscle and bone. Exposure to Galactic Cosmic Rays (GCR) and solar particle events raises cancer and degenerative health risks. By slashing transit times below one year, advanced nuclear propulsion would minimize these threats and reduce the need for massive radiation shielding.
Designing a crewed Titan mission also demands life‑support recycling, habitat modules, and emergency return options. Concepts borrow from Blue Origin’s lunar habitat studies and Mars transit designs, with shared technologies adapted for longer missions in deeper space.
Table 2: Health Risk Factors
Risk Factor | Mitigation via Nuclear Propulsion |
---|---|
Microgravity effects | Shorter transit reduces deconditioning |
Cosmic radiation dose | Faster transit lessens exposure |
Psychological stress | Reduced mission duration aids morale |
Future Prospects
The road to Titan requires advancing reactor safety, testing in Earth orbit, and international collaboration. Agencies like NASA, ESA, and private firms must conduct reactor demonstration missions beyond LEO. Partnerships with non‑profits like Explore Titan (https://exploretitan.org/) and academia (see Marcos Fernandez‑Tous at the University of North Dakota: https://campus.und.edu/directory/marcos.fernandeztous) bolster research and outreach.
By the 2040s, a fusion‑or fission‑powered ship could carry astronauts to Titan’s surface. There, they could study its vast seas of methane and possibly detect signs of simple life forms in this alien ocean world.
Facts
- Titan’s surface pressure is 1.5 times that of Earth’s, making flight easier for rotorcraft.
- Methane rain on Titan carves river channels just like water does on Earth.
- The Huygens probe reached Titan’s surface in January 2005, sending back the first images of its landscape.
References
- O’Hara, W. J. & Fernandez‑Tous, M. Nuclear Fission Propulsion System Sizing for a Greatly Accelerated Human‑Class Mission to Titan LPSC 2025 paper
- NASA SP‑2009‑566. Design Reference Architecture 5.0, Human Exploration of Mars (PDF)
- Copernicus NTP concept. NTRS (20110011201)
- Direct Fusion Drive study. Phys.org (DFD fusion engine for Titan)
- Dragonfly rotorcraft mission. Wikipedia (Dragonfly)
- NASA Dragonfly overview. NASA Science (Dragonfly mission page)
- Dragonfly nuclear battery detail. The Guardian (NASA to send Dragonfly drone to Titan)
- LPSC 2025 program. USRA (Technical program)
- University of North Dakota directory. UND (Marcos Fernandez‑Tous)
- Explore Titan, Inc. official site. (Explore Titan)
- Dragonfly mission details. NASA (Dragonfly nuclear‐powered rotorcraft)