Webb Observes Protoplanetary Disks that Contradict Models of Planet Formation
The James Webb Space Telescope (JWST) has unveiled groundbreaking insights into the longevity of protoplanetary disks in environments with low heavy-element content, challenging existing models of planet formation. Observations from the Small Magellanic Cloud (SMC) reveal that disks around young stars endure longer than previously thought, offering new perspectives on the formation of massive planets in the early universe.
Summary
- The James Webb Space Telescope (JWST) was designed to address fundamental cosmic questions such as galaxy formation, black hole origins, and planetary system evolution.
- Earlier models suggested that the early universe lacked sufficient heavy elements (metals) for the formation of massive planets.
- Hubble Space Telescope (HST) observations in 2003 identified a massive planet near an ancient star, defying these assumptions.
- Recent Webb observations of the Small Magellanic Cloud (SMC) revealed that stars in low-metallicity environments have longer-lived protoplanetary disks.
- Protoplanetary disks around stars in the SMC have lifespans of up to 20–30 million years, unlike the 2–3 million years typical in the Milky Way.
- This longevity suggests that planetary systems in metal-poor regions of the universe have more time to form.
- Two mechanisms may explain this phenomenon:
- Lower metallicity reduces the efficiency of stellar radiation in dispersing disks.
- Larger gas clouds in metal-poor environments result in more massive disks, which take longer to dissipate.
- Scientific implications include the need to revisit models of planet formation and early universe star formation.
- The findings reinforce JWST’s role in expanding our understanding of the cosmos, prompting new theories and discoveries.
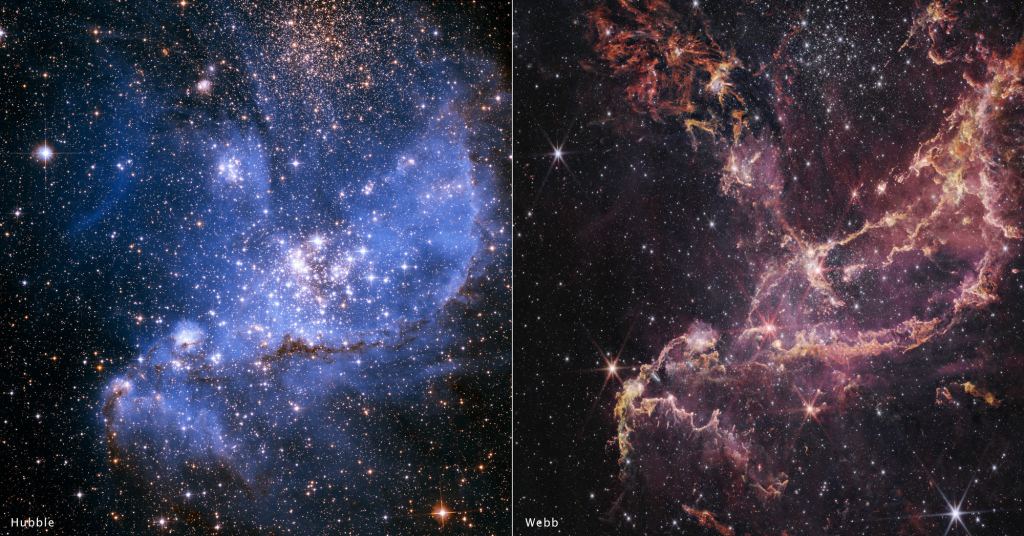
Protoplanetary Disks and the Evolution of Planets
Protoplanetary disks are the regions of gas and dust that surround young stars and are the birthplaces of planets. Understanding their lifespan and composition is critical for comprehending how planetary systems like our solar system formed. Previous assumptions suggested that such disks, especially in low-metallicity environments like the early universe, dissipated quickly due to radiation from their parent stars.
The Hubble Space Telescope’s (HST) discovery in 2003 of a massive Jupiter-like planet orbiting a star just a billion years after the Big Bang was a pivotal moment. It highlighted the possibility that planets could form earlier in the universe’s history than previously assumed.
Webb’s Observations of the Small Magellanic Cloud
The Small Magellanic Cloud (SMC) is a dwarf galaxy with only about 10% of the heavy elements found in the Milky Way. Its low metallicity mirrors the conditions of the early universe, making it an ideal laboratory for studying planet formation in environments with limited heavy elements.
JWST focused on NGC 346, a massive star cluster in the SMC, where young Sun-like stars were observed with protoplanetary disks. These disks defied conventional wisdom by lasting 20–30 million years, significantly longer than their Milky Way counterparts.
Mechanisms for Disk Longevity
The research team proposed two potential mechanisms to explain the extended lifetimes of these disks:
Mechanism | Explanation |
---|---|
Radiation Inefficiency in Low Metals | Radiation from stars is less effective at dispersing disks when there are fewer heavy elements. This allows disks in low-metallicity environments to persist longer. |
Larger Initial Disk Mass | Stars in metal-poor regions form from larger gas clouds, creating more massive disks. These disks require more time to dissipate, allowing extended planet formation. |
Redefining Planet Formation Models
JWST’s observations necessitate a significant revision of existing planet formation theories. The longevity of protoplanetary disks in environments with scarce heavy elements opens up new possibilities for planetary system architecture and evolution.
Elena Sabbi emphasized this paradigm shift:
“With more matter around the stars, the accretion lasts for a longer time. The disks take ten times longer to disappear. This has implications for how you form a planet and the type of system architecture that you can have in these different environments.”
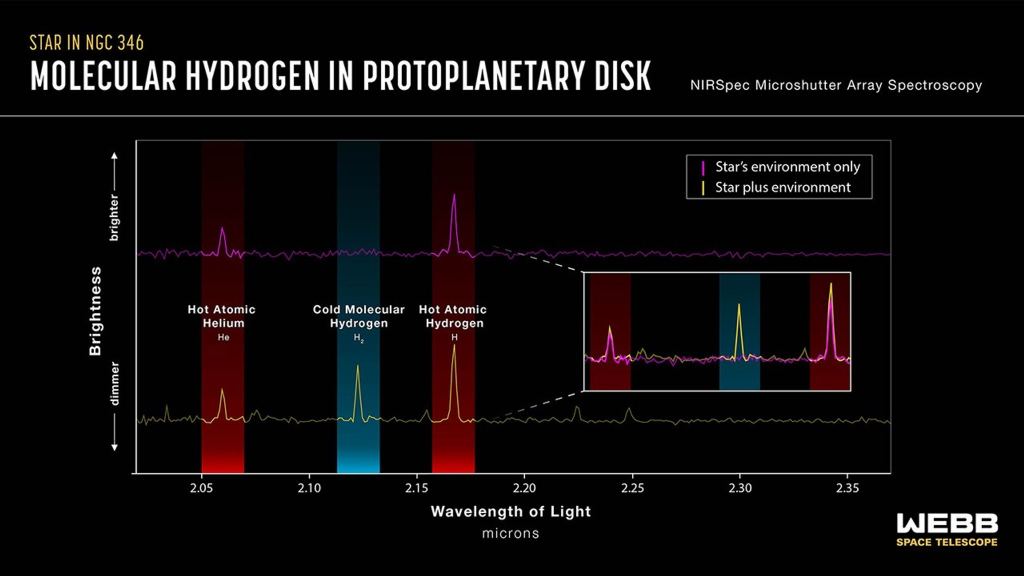
Comparison of Star-Forming Clusters
The insights gained from the SMC highlight significant differences between star-forming clusters in diverse environments. Below is a comparative table showcasing key distinctions:
Feature | Milky Way (High Metallicity) | Small Magellanic Cloud (Low Metallicity) |
---|---|---|
Disk Lifespan | 2–3 million years | 20–30 million years |
Heavy Element Content | High | Low |
Planet Formation | Faster | Slower but with extended growth periods |
Disk Mass | Moderate | Larger |
Implications for Cosmology
The discoveries in NGC 346 underscore the importance of reevaluating cosmological models. If protoplanetary disks persist longer in low-metallicity environments, it raises questions about the timeline of planet formation and the diversity of planetary systems across the universe.
JWST’s role in these revelations cannot be overstated. By challenging long-standing theories, it has provided a window into the early universe that was previously unattainable. Guido De Marchi, the study’s lead author, remarked:
“With Webb, we have a really strong confirmation of what we saw with Hubble, and we must rethink how we model planet formation and early evolution in the young universe.”
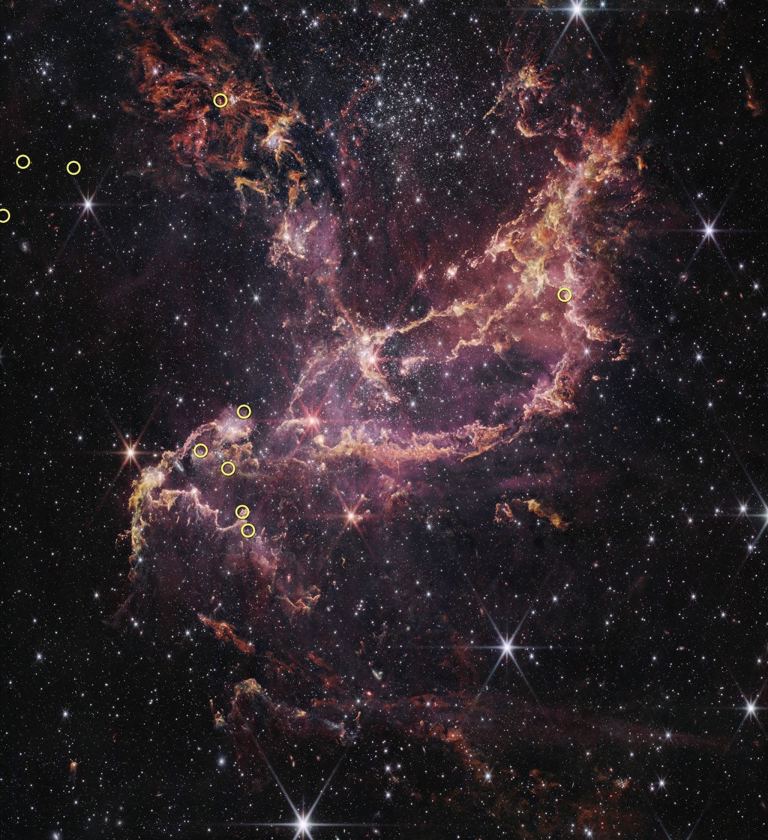
Facts About JWST
- JWST is 100 times more powerful than Hubble, allowing it to peer into the early universe with unprecedented clarity.
- It operates primarily in the infrared spectrum, making it ideal for studying cold objects like protoplanetary disks.
- JWST’s instruments include NIRCam, MIRI, NIRSpec, and FGS/NIRISS, each specialized for specific observations.
The James Webb Space Telescope continues to redefine our understanding of the cosmos. By observing protoplanetary disks in the Small Magellanic Cloud, it has uncovered evidence that challenges existing theories of planet formation. These findings not only highlight the complexity of cosmic evolution but also pave the way for future discoveries that could reshape our knowledge of the universe.
For further insights, explore the following resources:
References
- NASA. “James Webb Finds Planet-Forming Disks Lived Longer in Early Universe.” Link
- The Astrophysical Journal. “Protoplanetary Disks in the Small Magellanic Cloud.” Link
- European Space Agency. “Webb Observations of NGC 346.” Link
- NOIRLab. “Insights from Gemini Observatory.” Link
- UK Astronomy Technology Centre. “Research on Star Formation.” Link